Grain Size and Depositional Environment
There are four primary ways to estimate depositional environment
from well logs:
1. shale volume/grain size analysis
2. dip spread/water depth analysis
3. bedding angle/bedding type analysis
4. curve shape/depositional sequence analysis
All
of the techniques rely on a strong correlation between depositional
environment and the energy needed to produce certain characteristics
that we can see on well logs over the rock sequence.
Depositional
energy level correlates well to grain size, which in turn is usually
proportional to shale volume. Thus the gamma ray or SP curve can
augment environment estimates from dipmeter analysis. Low values
of gamma ray (or high SP) indicate high energy, low shale content
zones. These are inner shelf or upper continental slope in a marine
environment, or alluvial or fluvial regimes on the continent.
Higher
shale volume indicates lower energy deposition; that is, deeper
water outer shelf, lower continental slope, continental lacrustine,
or paludal environments.
Curve
shape analysis depends almost entirely on the shape of the SP
or GR curves versus depth, so the shale volume/grain size/depositional
energy relationship is a strong component of our analysis method.
The reconstructed resistivity curve from the dipmeter or a microresistivity
curve can also be used as a grain size indicator in shaly sand
sequences. An example is shown below, from a SYNDIP presentation.
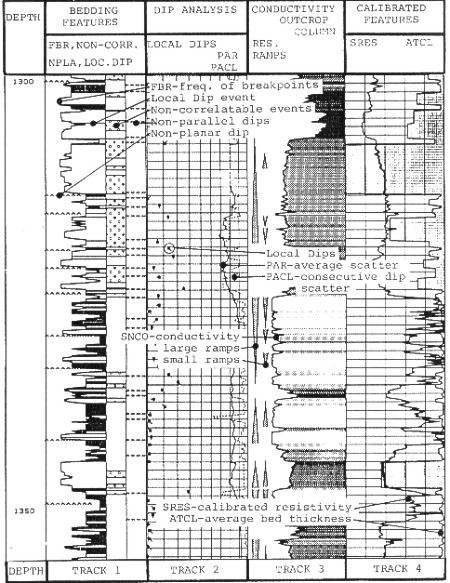
Grain size estimates from log curves (SYNDIP)
A combination of curve shape and bedding patterns are used to
differentiate the ambiguity obvious in the above discussion. Grain
size alone, as indicated by shale content, is not a sufficient
criteria to determine the environment, but it does help distinguish
high, medium, and low energy environments.
Dip Spread and Depositional Environment
For most situations, the spread in the dip angle values correlates
to energy level, as shown below.
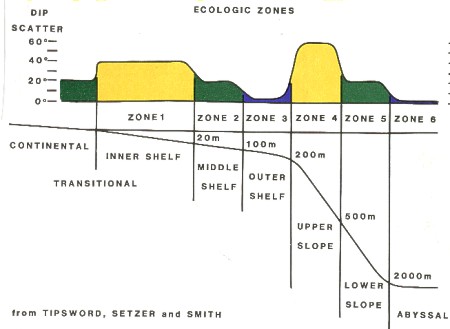
Depositional environment, water depth, and dip
scatter
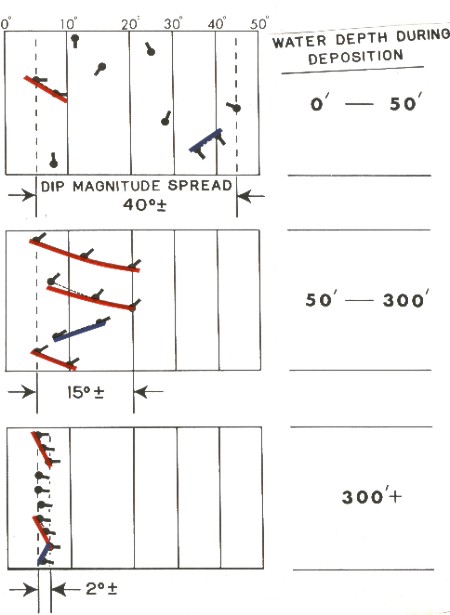
Dip scatter and water depth
The
continental slope and abyssal plain zones also have distinctive
energy patterns, with very high energy at the upper slope, due
to slumping and turbidity currents. Energy levels decrease rapidly
with distance from the upper slope. This results in dip ranges
of 60 degrees in the upper slope zone to a few degrees in the
abyss, corresponding to Zones 4, 5, and 6.
Dips
on the continental zone range up to 20 degrees for fluvial deposits
and 45 degrees for eolian and alluvial deposits.
Dip
Spread in Various Depositional Environments
Zone |
Energy |
Features |
Grain |
Dip
Range |
Water
Depth |
|
Level |
|
Size |
degrees |
feet |
|
|
|
|
|
|
Continental |
High |
Scree
slopes |
V.
Coarse |
20
- 45 |
0
- 50 |
Zone
0 |
|
Alluvial
fans |
Coarse |
0
- 30 |
|
|
Medium |
Braided
stream |
Medium |
0
- 20 |
|
|
|
Point
bars |
Fine |
0
- 20 |
|
|
Low |
Channel
fill |
V.Fine |
0
- 10 |
|
|
High |
Glacial
till |
Mixed |
scattered |
|
|
|
Eolian
dunes |
Coarse |
10
- 45 |
|
|
|
|
|
|
|
Inner
Shelf |
High |
Sand
bars |
Coarse |
0
- 30 |
0
- 50 |
Zone
1 |
|
Tidal
channels |
Medium |
0
- 25 |
|
|
|
Ebb
deltas |
|
0
- 25 |
|
|
|
Flood
deltas |
|
0
- 20 |
|
|
|
Washover
fans |
|
0
- 20 |
|
|
|
|
|
|
|
Middle
Shelf |
Medium |
Distributary |
Medium |
0
- 15 |
50
- 300 |
Zone
2 |
|
channels |
|
|
|
|
|
Longshore
current |
|
0
- 15 |
|
|
|
channels |
|
|
|
|
|
Distributary |
Coarse |
0
- 20 |
|
|
|
fronts |
|
|
|
|
|
|
|
|
|
Outer
Shelf |
Low |
Distributary |
Fine |
0
- 5 |
300
- 600 |
Zone
3 |
|
channels |
|
|
|
|
|
Longshore
current |
|
0
- 5 |
|
|
|
channels |
|
|
|
|
|
Distributary |
Medium |
0
- 5 |
|
|
|
fronts |
|
|
|
|
|
|
|
|
|
Upper
Slope |
High |
Slide
cut channels |
Coarse |
0
- 60 |
600
- 1000 |
Zone
4 |
|
Turbidite
fans |
|
0
- 60 |
|
|
|
|
|
|
|
Lower
Slope |
Medium |
Slide
cut channels |
Medium |
0
- 25 |
1000
- 3000 |
Zone
5 |
|
Turbidite
fans |
|
0
- 25 |
|
|
|
|
|
|
|
Abyssal
Plain |
Low |
Turbidite
Fans |
Fine |
0
- 5 |
3000+ |
Zone
6 |
|
|
|
|
|
|
|
|
|
|
|
|
|
Even
though energy level, water depth, and grain size can be inferred
from logs, this is still not enough information to segregate all
sedimentary structures.
Current
Bedding and Depositional Environment
The geometry of a sandstone unit is related to its internal structures,
which are functions of its depositional environment. The internal
structure is influenced mostly by current bedding. The direction
of paleocurrents is indicated by the orientation of the current
bedding, which can be measured by using dipmeter data after removal
of structural dip. This direction is an aid to tracking the reservoir,
but the correct model for the reservoir must be chosen before
the direction information is of any use.
The
term current bedding is used to describe the beds laid down in
a channel parallel to the direction of current flow. The current
beds will dip downward in the direction of the current flow and
will be from a few inches to a few feet thick.
Crossbedding,
although the term suggests otherwise, is also parallel to the
direction of current flow. However, crossbeds do not often occur
in river channels but usually on the front of deltas or shallow
marine sand bars. Crossbeds dip considerably more steeply, but
in the same direction, as the dips of the delta or sand bar surface.
Planar or tabular bedding, as the words suggest, involve flat
layers of rock (maybe lying at an angle) laid down in streams,
lakes, or in deltas. Festoon bedding creates layers which are
convex top and bottom, and are usually laid down in braided
streams. Wedge shaped or nonparallel bedding is planar bedding
with concurrent erosion which has removed a portion of the bed,
such as on the curve of a meandering river. Examples of these
bedding patterns are given below.
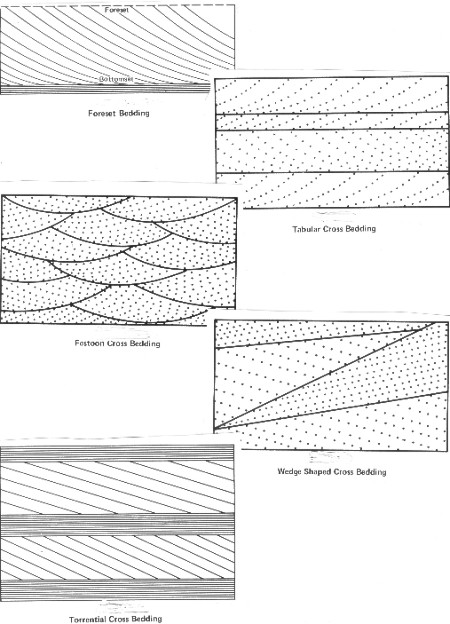
Bedding patterns
As
described earlier, a strong correlation exists between depositional
energy and grain size of the rocks. The larger the grain size,
the greater the depositional energy. Therefore, steep current
bedding, which can only be supported by large grains, is usually
interpreted as high energy deposition. Flatter beds represent
lower energy deposition. This rule usually holds when deposition
occurs in a place away from the transportation artery, such as
in a delta front or when deposition is associated with ocean wave
energy.
However,
this rule could be broken when deposition and transportation occur
simultaneously, as in a channel, where the highest energy may
produce the flattest, even reversed, current bedding dips.
Specific
sedimentary environments give rise to characteristic patterns
of current bedding dips versus depth. Such patterns, seen on the
dipmeter plot, can be used to help identify the depositional environment.
For example, most bar type deposits will exhibit a high dip angle
in the upper part, decreasing to a low angle near the base.
Bedding
Characteristics in Various Depositional Environments
|
|
|
Depositional |
Current
Bedding |
Current
Bedding |
Environment |
Characteristics |
Orientation |
|
|
|
Glacial
deposits |
None
or very fine varves |
Non
or down paleoslope |
|
|
Direction
of sand elongation |
|
|
|
Braided
stream |
Festoon
(trough) type |
Unimodal
large scatter |
alluvium |
Steep
dip |
Generally
down pateoslope |
|
|
Direction
of sand elongation |
|
|
|
Meandering
stream |
Festoon
(trough) type |
Unimodal
- severe scatter |
point
bars |
Large
dip spread |
Generally
down paleoslope |
|
Higher
angle at base |
Direction
of meander belt and |
|
Low
angle tabular at top |
sand
body alignment |
|
|
|
Eolian
dunes |
Tabular
- high angle |
Little
scatter |
|
Extremely
consistent |
No
relation to paleoslope |
|
Decreasing
angle at base |
Normal
to sand elongation |
|
|
|
Delta
distributary |
Festoon
- tabular |
Unimodal
- moderate scatter |
channels |
Higher
angle at base |
In
seaward direction |
|
Moderate
spread |
Direction
of sand elongation |
|
|
|
Distributary
mouth |
Tabular
moderate angle |
Unimodal
- radiation |
bars |
(>10
degrees) |
seaward
direction but |
influenced |
|
|
|
Higher
angle at top |
by
longshore currents |
|
Moderate
spread |
Direction
of sand elongation |
|
(Lobate) |
|
|
|
|
Estuarine
& tidal |
Tabular
- low angle |
Bimodal
(180 deg) - |
scattered |
|
|
channels |
(<10
degrees) |
Normal
to coastline |
|
Higher
angle at base |
Direction
of sand |
elongation |
|
|
|
Flatter
at top |
|
|
|
|
Beaches
and bars |
Tabular |
Unimodal
- possibly bimodal |
|
Low
angle on seaward |
Usually
down paleoslope |
but |
|
|
|
Side
(<10 deg) |
possibly
reversed |
|
High
angle on lagoonal |
Normal
to sand elongation |
|
side
(<20 deg) |
|
|
|
|
Marine
shelf |
Tabular |
Polymodal
- random sands |
|
Very
low angle throughout |
|
|
|
|
Turbidites |
Tabular
or absent |
Unimodal |
|
Very
low angle throughout |
Down
paleoslope |
|
Rarely
observable |
Direction
of sand elongation |
|
|
|
|
|
|
|
This
table is adapted from "Reservoir Delineation By Wireline
Techniques" by J.F.Goetz, W.J.Prins, and J.F.Logan, published
in The Log Analyst, June, 1977.
To
evaluate current bedding, its characteristics (type, angle, pattern,
spread) and its orientation (direction and scatter) are considered
together. The above tables should be used in conjunction with
the sedimentary model descriptions given later in this Chapter.
In
case of a conflict between evidence supplied by the various approaches,
current bedding patterns should overrule curve shapes, because
the dipmeter has better resolution. This extends to the determination
of the boundaries of genetic units. Sometimes the incoming material
may change while the same depositional conditions persist, with
the result that lithological unit boundaries may not match those
of genetic units. One genetic unit may be made up of more than
one lithological unit or vice versa. Interpretation involving
sedimentary structures is based on genetic units and should not
be too strongly influenced by lithology variations.
Curve Shape Analysis and Depositional Environment
Grain size and bedding both influence the overall curve shape
of a log versus depth. There are four basic curve shapes:
1.
straight line, indicating constant shale, evaporite, clean sand,
or carbonate, caused by continuous deep water deposition
2.
bell shaped, indicating a fining upward sequence, ie., lower energy
at the end of a cycle
3.
funnel shaped, indicating a coarsening upward sequence, ie higher
energy at the end of a cycle
4.
cylindrical shaped, indicating constant energy throughout the
cycle
The
last three are the usual patterns considered in an environment
analysis. Variations exist. Serrated patterns are caused by abrupt
changes in energy, resulting in layers of silt or shale interbedded
in an otherwise regular pattern. Short patterns way be imbedded
in longer ones. Thus, short coarsening upward patterns may contribute
to a larger coarsening upward pattern. Patterns of all three kinds
may be imbedded in one larger one.
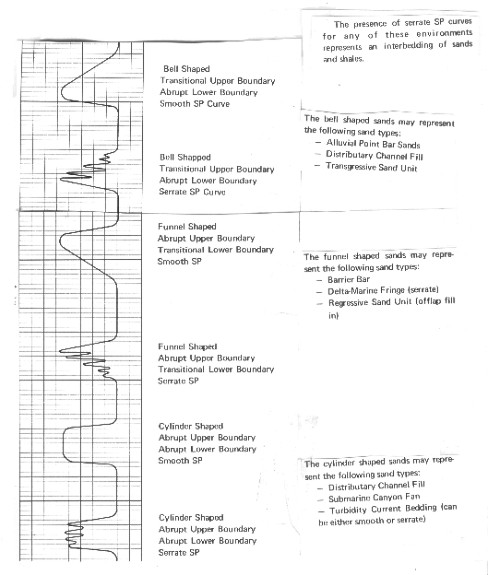
Fining upward, coarsening upward, and cylindrical
curve shape
Curve
Shapes in Various Depositional Environments
|
|
|
Curve
Shape |
|
|
Pattern |
Characteristics |
Represents |
Bell |
Transitional
upper boundary |
Alluvial
point bar sands |
|
Abrupt
lower boundary |
Distributary
channel fill |
|
Smooth
or serrated |
Transgressive
marine sand |
|
|
Drape
over reef |
|
|
Drape
inside channel |
|
|
Tidal
channel |
|
|
|
Funnel |
Abrupt
upper boundary |
Barrier
bar |
|
Transitional
lower boundary |
Delta
front |
|
Smooth
or serrated |
Regressive
marine sand |
|
|
Crossbedding |
|
|
Foreset
bedding |
|
|
Distributary
front |
|
|
Distributary
mouth bar |
|
|
|
Cylinder |
Abrupt
top and bottom |
Distributary
channel fill |
|
Smooth
or serrated |
Turbidite
fan |
|
|
Submarine
canyon fan |
|
|
Eolian
Dunes |
|
|
|
Funnel
- Bell |
Transitional
top and bottom |
Marine
shelf sand |
|
|
Turbidite |
|
|
|
|
|
|
Straight
line |
No
character |
Deep
water continuous |
|
|
deposition |
|
|
Carbonate
bank |
|
|
Marine
shale |
|
|
|
|
|
|
|
These shapes are most obvious on gamma ray and SP curves, but
may also be derived from resistivity (on a logarithmic scale),
porosity, or a computed curve. In particular, the synthetic resistivity
curve on the dipmeter arrow plot or SYNDIP presentation are widely
used.
INTEGRATED APPROACHES TO DEPOSITIONAL: ENVIRONMENT
Combining
the rules for grain size indicators, dip spread, current bedding,
and curve shape is a formidable task. Add to this the palynology
and paleontology (micro and macro fossils), as well as the lithology
descriptions, and you have an almost undecipherable problem to
solve. A rule based expert system could be constructed from the
tables given above. An example is shown below, from
a USGS program.
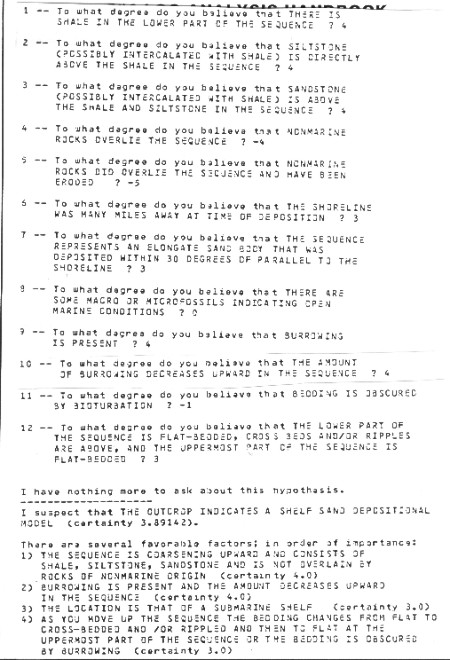
USGS expert system to determine depositional environment
|