Igneous
and Metamorphic Basics
Medium temperature
geothermal projects are often placed in sedimentary rocks,
where log analysis methods and rock properties are
reasonably well understood. High temperature geothermal is
more common in igneous and metamorphic rocks. These are more
difficult for petrophysicists to analyze as the physical
properties are more variable than those for sedimentary
minerals.
Further, igneous
and metamorphic rocks are often described by a rock-type
name and not by their mineral content. Since logs respond to
minerals and not rock-types, an extra step is required to
generate rock-types from the mineral components.
This article
describes the rock properties as seen by well logs, and the
mineral composition of the common rock-types. The objective
is to provide the data needed so that you can use your
favourite multi-mineral model to resolve the mineralogy of a
potential geothermal reservoir in non-sedimentary settings.
Metamorphic rock classification
Metamorphic rocks are conventional sedimentary rocks that have
been exposed to high heat and pressure. There are several types
of metamorphism: contact, regional, hydrothermal, or fault zone
friction,
Changes that occur during metamorphism are re-crystallization,
neomorphism in which new minerals are created from the original,
and metamorphism in which new minerals are created by gaining or
losing chemical elements.
Specific sedimentary rocks become specific metamorphic rocks, as
shown below:
Sandstone
č
Quartzite
Limestone OR Dolomite
č
Marble
Basalt
č
Schist OR Amphibolite
Shale
č
Slate
Granite OR Rhyolite
č
Schist
These names are familiar to most geologists, but not to many
engineers and log analysts who grew up in a sedimentary world.
METAMORPHIC ROCK
PROPERTIES
The petrophysical properties of metamorphic rocks are often
similar to their pre-metamorphic sedimentary counterparts as
long as different minerals have not formed. Standard 2- and
3-mineral models, or probabilistic multi-mineral models, are
used to calculate lithology. The density neutron complex
lithology model is used to calculate porosity when data and
borehole conditions permit. Sonic neutron crossplot models can
be used as an alternate when needed.
All the algorithms needed are coded in most petrophysical
software packages. For explanations of the math, see Reference
1. See Table 1 at the end of this article for a list of matrix
properties for metamorphic rocks.
MATRIX PROPERTIES FOR METAMORPHIC MINERALS |
|
DENSMA
g/cc |
DTCMA
usec/ft |
PHINMA
frac |
PE |
Plith |
Mlith |
Nlith |
Quarzite |
2.65 |
55.5 |
-0.028 |
1.82 |
1.174 |
0.861 |
0.663 |
Lime Marble |
2.71 |
47.3 |
0.000 |
6.09 |
3.161 |
0.880 |
0.621 |
Dolo Marble |
2.90 |
43.9 |
0.040 |
3.13 |
1.759 |
0.819 |
0.562 |
Slate |
3.15 |
60.0 |
-0.030 |
3.55 |
? |
? |
? |
Granite Schist |
2.66 |
55.0 |
0.000 |
1.88 |
1.174 |
0.861 |
0.663 |
Igneous rock classification
Most people think of granite or lava flows when igneous rocks
are mentioned If only it was that simple. There are many
variations in rock properties and rock types to take into
account during a petrophysical analysis. The mineral and
porosity models needed are the same as noted earlier for
metamorphic rocks It is more challenging because geologists
describe rock-types, which are variable mixtures of minerals,
while logs respond only to minerals and not rock-types. We will
show how to fix that later on in this article.
Igneous rocks are classified in several ways – by composition,
texture, and method of emplacement. The composition (mineral
mixture and internal porosity) determines the log response. The
texture determines the name used for the mineral mixture, and
the method of emplacement determines the texture and internal
porosity structure (if any). The same mineral mixture can have
more than one name based on its crystal size and method of
emplacement.
Intrusive igneous rocks are formed inside the earth. This type
cools very slowly and is produced by magma from the interior of
the earth. They have large grains, may contain gas pockets, and
usually have a high fraction of silicate minerals. Intrusions
are called sills when lying roughly horizontal and dikes when
near vertical.
Extrusive igneous rocks form on the surface of the earth from
lava flows. These cool quickly. They have small grains and
contain little to no gas.
Both intrusive and extrusive rocks may contain natural fractures
from contraction while cooling, and may have carried non-igneous
rocks with them, called xenoliths.
Intrusive rocks may alter the rocks above and below them by
metamorphosing (baking) the rock near the intrusion. Extrusives
only heat the rock below them, and may not cause much
iteration due to rapid cooling. Extrusives can be buried by
later sedimentation, and are difficult to distinguish from
intrusives, except by their chemical composition and grain size.
The mineral composition of an igneous rock depends on where and
how the rock was formed. Magmas around the world have different
mineral make up.
Felsic igneous rocks are light in color and are mostly made up
of feldspars and silicates. Common minerals found in felsic rock
include quartz, plagioclase, feldspar, potassium feldspar
(orthoclase), and muscovite. They may contain up to 15% mafic
mineral crystals and have a low density.
Mafic igneous rocks are dark colored and consist mainly of
magnesium and iron. Common minerals found in mafic rocks include
olivine, pyroxene, amphibole, and biotite. They contain about
46-85% mafic mineral crystals and have a high density.
Ultramafic igneous rocks are very dark colored and contain
higher amounts of the same common minerals as mafic rocks, about
86-100% mafic mineral crystals.
Intermediate igneous rocks are between light and dark colored.
They share minerals with both felsic and mafic rocks. They
contain 15 to 45% mafic minerals.
Plutonic and volcanic rocks generally have very low porosity and
permeability. Natural fractures may enhance porosity by allowing
solution of feldspar grains.
Tuffs and tuffaceous rocks have high total porosity because of
vugs or vesicles in a glassy matrix. This is most common in
pyroclastic deposits. Interparticle porosity may also exist.
Some effort has to be made to separate ineffective microporosity
from the total porosity. Pumice (a form of tuff) has enough
ineffective porosity to allow the rock to float on water! When
other minerals fill the vesicles by precipitation, the tuff is
called a zeolite.
IGNEOUS ROCK CLASSIFICATION |
Plutonic |
Volcanic |
Pyroclastic |
|
Gamma Ray |
Coarse Crystalline |
Fine
Crystalline |
Glassy |
Silica Content |
Density |
Quartzite |
|
|
Highest |
Lowest |
Granite |
Rhyolite |
Rhyolite Tuff |
|
|
Granodioite |
Dacite |
Dacite Tuff |
|
|
Quartzdiorite |
Andesite |
Andesite Tuff |
|
|
Diorite |
Basalt |
Zeolite Tuff |
|
|
Gabbro |
Dolerite |
|
|
|
Disabase |
|
|
|
|
Dunite |
|
|
Lowest |
Highest |
|
For quick-look identification of igneous rocks, crossplots have been
widely used for many years. Before the advent of the PE curve,
crossplots using neutron, sonic and density were the best bet. Some
prior calculations are required. Matrix density (DENSma), sonic
matrix travel time (DTCma), lithology factors Mlith and Nlith must
be derived. With the PE curve, a lithology factor called Plith can
be added, as well as Uma, the matrix capture cross section. Examples
are shown below.
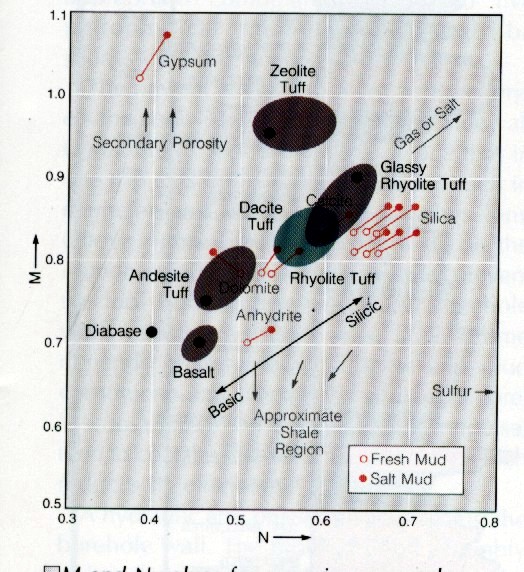
DENSma vs DTCma Crossplot
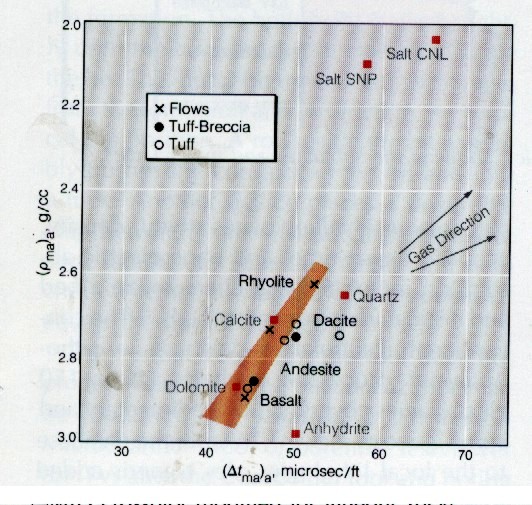
Mlith vs Nlith Crossplot
Igneous MINERAL properties
Most igneous rocks are described by their rock-type and not by
their mineral composition. For example, granite is a rock-type
composed of the minerals quartz, feldspar, and plagioclase. Logs
respond to the mineral mixture, not the rock-type. Once the
mineral fractions are derived by a suitable log analysis model,
a second step is needed to convert the minerals to rock-types.
Properties for individual minerals are better known and less
variable than rock-type values. It is more accurate to use a
mineral model than a rock-type model. Here are the mineral
properties that can be used in the various multi-mineral log
analysis models. These are the same values that might be used in
a sedimentary rock sequence, sorted to reflect the common
constituents of igneous rocks.
MATRIX PROPERTIES FOR IGNEOUS MINERALS |
|
DENSMA
g/cc |
DTCMA
usec/ft |
PHINMA
frac |
PE |
Plith |
Mlith |
Nlith |
Magnetite |
5.08 |
73.0 |
0.0 |
22.0 |
5.3922 |
0.2794 |
0.2451 |
Hornblend |
3.20 |
43.8 |
0.0 |
6.0 |
2.7273 |
0.6509 |
0.4545 |
Quartz |
2.64 |
56.0 |
-0.02 |
1.8 |
1.0976 |
0.7988 |
0.6098 |
K Feldspar |
2.52 |
46.0 |
-0.03 |
2.9 |
1.9079 |
0.9276 |
0.6579 |
Plagioclase |
2.62 |
53.0 |
0.0 |
3.0 |
1.8519 |
0.8272 |
0.6173 |
Biotite |
3.00 |
55.0 |
0.21 |
6.3 |
3.1500 |
0.6800 |
0.4990 |
Pyrite |
4.99 |
39.2 |
0.06 |
17.0 |
4.2607 |
0.3704 |
0.2505 |
Sometimes a mineral is determined by triggers based on their
specific log responses. For example, where basalt beds are
interspersed between conventional granite or quartzite, it is easy
to use the PE or density logs to trigger 100% basalt, leaving the
remaining minerals to be defined by a two or three mineral model.
CONVERTING minerals TO ROCK-TYPES
After determining the mineral composition, the rock-type can be
estimated from a near-fit to the mineral composition shown in
the table below.
CONVERTING MINERALS TO ROCK-TYPES |
|
Granite |
GranoDiorite |
QuartzDiorite |
Diorite |
Gabbro |
Plagioclase |
0.30 |
0.46 |
0.53 |
0.63 |
0.53 |
Quartz |
0.27 |
0.21 |
0.22 |
0.02 |
0.00 |
K Feldspar |
0.35 |
0.15 |
0.05 |
0.03 |
0.16 |
Orthopyroxene |
0.00 |
0.00 |
0.00 |
0.00 |
0.15 |
Other |
0.08 |
0.18 |
0.20 |
0.32 |
0.16 |
This table is based on the illustration given below, courtesy of
Schlumberger.
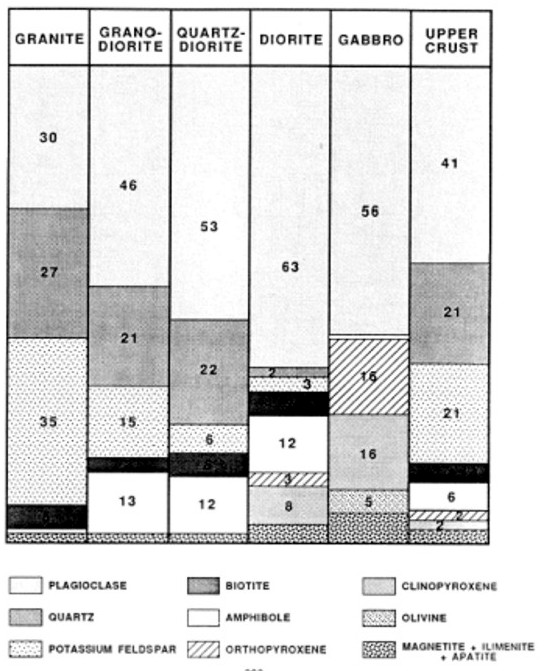
Typical mineral composition of igneous rocks – use as a guide to
convert minerals to rock-types.
Since a typical log suite can solve for 3 or 4 minerals at best, you
need to choose the dominant minerals and zone your work carefully.
If you have additional useful log curves, you might try for more
minerals or set up several 4 mineral models in a probabilistic
solution. A good core or sample description will help you choose a
reasonable mineral suite.
eXAMPLe #1 - METAMORPHIC SAND / GRANITE
Here
is a granite/metamorphic example from Indonesia. The reservoir
has a porous granite at the base, metamorphic sandstone above,
topped by conventional sandstone. Porosity is moderately low
throughout but the gas column is continuous. Interbedded shales
(schist or gneiss in the metamorphic interval) are present but do
not act as barriers to vertical flow.
In this case, the mineralogy
was calibrated by quantitative sample descriptions, which in turn
were keyed to raw log response to minimize cavings and depth control
issues. Porosity and water saturation were derived from
conventional log analysis methods. The reservoir is naturally
fractured and a fracture intensity curve was generated from
anomalies on the open hole logs. This was compared to the fracture
intensity from resistivity micro image log data.
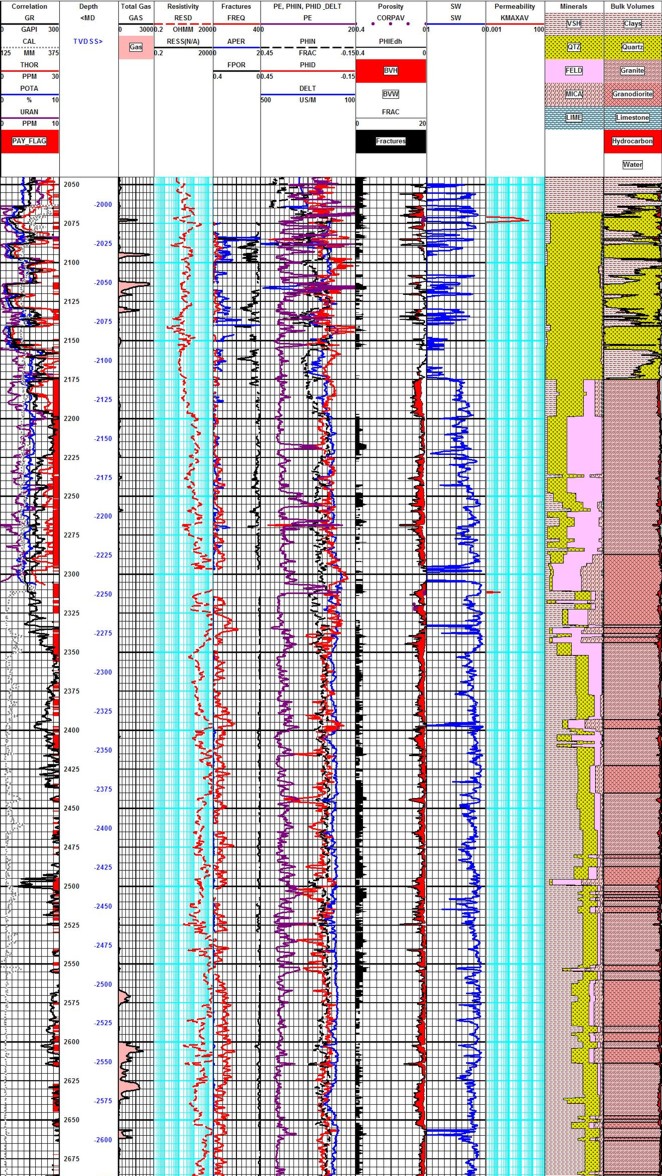
Metamorphic / Granite example with spectral GR (Track 1), total
gas (Track 2), resistivity (Track 3), fracture aperture, fracture
intensity, fracture porosity (from FMI processing, Track 4),
density, neutron, PE (Track 5), log analysis porosity (Track 6),
water saturation (Track 7), core permeability (Track 8), quantitative sample
description (Track 9), calculated lithology (Track 10).
Compare fracture intensity from log anomalies (black shaded
curve in porosity track with fracture intensity from FMI (red curve,
track 4). Best gas production in granite is confirmed by gas show on
gas log and by production logging in open hole. Sample descriptions
show minerals as seen in microscope (quartz, feldspar, mica) to
nearest 5%. Log analysis lithology show rock type, not minerals
(quartz, granite, granodiorite). The sands and shale immediately
above the granite are metamorphosed, visible in samples, but there
is little effect on log properties except for low clay bound water
on neutron and density logs in the shale/slate. Some wells had
limestone marble in the metamorphosed interval.
eXAMPLe #2 -
FRACTURED GRANITE WITH POROSITY
Most people forget that there are many unconventional reservoirs
in the world, including igneous, metamorphic, and volcanic rocks.
Granite reservoirs are prolific in Viet Nam, Libya, and Indonesia.
Lesser known granite reservoirs exist in Venezuela, United States,
Russia, and elsewhere. Indonesia is blessed with a combination
sedimentary, metamorphic, and granite reservoir with a single
gas leg. Japan boasts a variety of volcanic reservoirs.
This
example is from the Bach Ho (White Tiger) Field in Viet Nam.
Log
analysis in these reservoirs requires good geological input as
to mineralogy, oil or gas shows, and porosity. A good coring and
sample description program is essential, and production tests
are essential. The analyst often has to separate ineffective
(disconnected vugs) from effective porosity and account for fracture
porosity and permeability. All the usual mineral identification
crossplots are useful but the mineral mix may be very different
than normal reservoirs. Many such reservoirs seem to have no water
zone and most have unusual electrical properties (A, M, N), so
capillary pressure data is usually needed to calibrate water saturation.
Ternary Diagram for Granite

In
the example below, the granitic mineral assemblage was defined by the ternary
diagram at right. The three minerals (quartz, feldspar,
and plagioclase) were computed from a modified Mlith vs Nlith
model, in which PE was substituted for PHIN in the Nlith equation.
If data fell too far outside the triangle, mica was exchanged
for the quartz.
Three
rock types, granite, diorite, and monzonite, were derived from
the three minerals. A trigger was set to detect basalt intrusions.
A sample crossplot below shows how the lithology model effectively separates
the minerals.
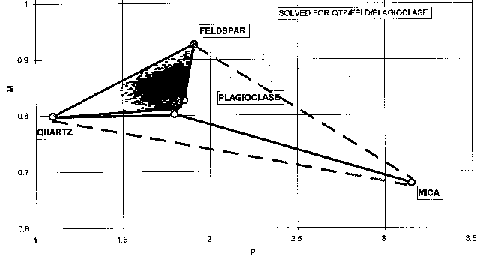
Mlith vs Plith crossplot for granite (micaceous
data excluded)
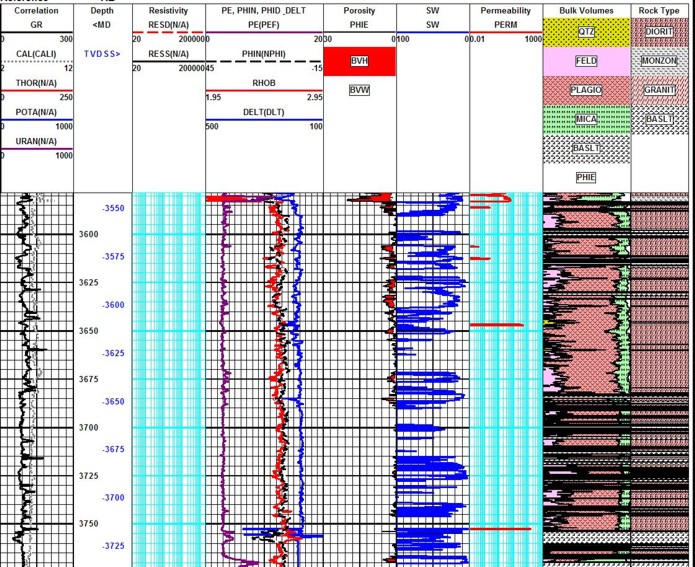
In this fractured granite example, raw data curves are shown in
Tracks 1, 2, and 3 with effective porosity, water saturation, and
matrix permeability in Tracks 4, 5, and 6. The mineral model
calculated from the log analysis is in Track 7 and the rock type
model calculated from the minerals using the ternary diagram is in
Track 8. Basalt was triggered from high density or high PE or both.
A
sample of the log analysis plot is shown above. The average porosity
from core and logs is only 0.018 (1.8%) and matrix permeability
is only 0.05 md. However, solution porosity related to fractures
can reach 17% and permeability can easily reach higher than several
Darcies. Customized formulae were devised to estimate these properties
from logs, based on core and test data. My colleague Bill Clow
devised most of the methods used on this project.
Fracture porosity from resistivity micro scanner logs
was also computed where available to help control the open hole
work. A black and white resistivity image log below shows
some of the fractures. Both high and low angle fractures co-exist.
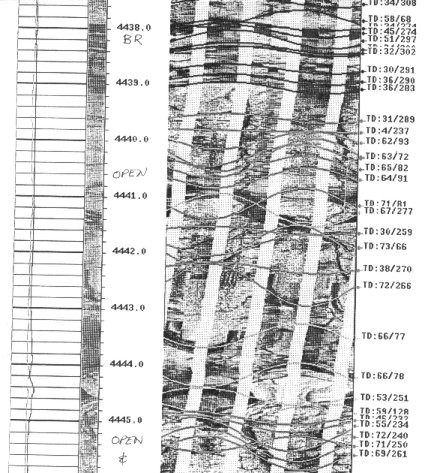
Resistivity micro scanner image in granite reservoir
It
is clear that non-conventional reservoirs may need some extra
effort, customized models, and unique presentations. Everything
you need to develop these techniques can be found elsewhere in this Handbook.
The mineral properties need to be chosen carefully, but the
mathematical models don't change too much.
|