Petrophysical Training
Licenses
|
NON-METALLIC MINERAL basicS
This article
covers petrophysical analysis of economically significant
non-metallic sedimentary mineral such as halite, gypsum,
trona, and sulphur. These minerals may be exploited for
their commercial value, or may be encountered while drilling
for oil and gas. A characteristic of these minerals is their
low density, very high resistivity, and very low gamma ray
response. Some have near zero neutron porosity response, but
others have water of hydration with a corresponding specific
neutron response.
These characteristics can be comfused with
high porosity hydrocarbon zones on a cursory visual analysis.
Lithology triggers are needed for
these minerals in petrophysical software to prevent erroneous
results.
Potash
minerals
(sylvite, carnalite, langbeinite, polyhalite, kainite) and
Coal
analysis
are covered elsewhere in this Handbook.
Halite, gypsum, and trona
occur as relatively pure bedded minerals, thick enough to be
resolved by well logs. These can be identified by their
specific mineral properties as listed in the tables below,
using lithology triggers.
Alternatively, the mineral properties can be used in 2- or
3-mineral models, simultaneous equations, or multi-mineral
probabilistic models.
In sulphur bearing rocks, lithology triggers will not work and
the more sophisticated methods must be used. Crossplots,
shown below the table of mineral properties, may also be
helpful in confirming or eliminating possibilities.
NON-METALLIC MINERAL
PROPERTIES -- FRESH MUD |
Mineral |
PHIN |
DENS |
DTC |
DTC |
PE |
Uma |
Mlith |
Nlith |
Alith |
Klith |
Plith |
|
Ls |
g/cc |
us/m |
us/ft |
barns |
cu |
frac |
frac |
frac |
frac |
frac |
Water |
1.000 |
1.00 |
656 |
200 |
0.10 |
0.10 |
|
|
|
|
|
|
|
|
Anhydrite |
0.002 |
2.95 |
164 |
50.0 |
5.08 |
14.99 |
0.769 |
0.512 |
1.954 |
1.503 |
2.605 |
Halite |
-0.010 |
2.03 |
220 |
67.1 |
4.72 |
9.58 |
1.291 |
0.981 |
1.020 |
1.316 |
4.583 |
Gypsum |
0.490 |
2.35 |
172 |
52.4 |
4.04 |
9.49 |
1.093 |
0.378 |
2.647 |
2.894 |
2.993 |
Trona |
0.350 |
2.08 |
213.3 |
65.0 |
0.71 |
1.48 |
1.250 |
0.602 |
1.662 |
2.077 |
0.657 |
Sulphur |
0.020 |
2.02 |
400.3 |
122.0 |
5.05 |
10.20 |
0.765 |
0.961 |
1.041 |
0.796 |
4.951 |
NON-METALLIC MINERAL
PROPERTIES -- SALTY MUD |
Mineral |
PHIN |
DENS |
DTC |
DTC |
PE |
Uma |
Mlith |
Nlith |
Alith |
Klith |
Plith |
|
Ls |
g/cc |
us/m |
us/ft |
barns |
cu |
frac |
frac |
frac |
frac |
frac |
Water |
1.076 |
1.10 |
620 |
189 |
0.10 |
0.11 |
|
|
|
|
|
|
|
|
Anhydrite |
0.002 |
2.95 |
164 |
50.0 |
5.08 |
14.99 |
0.751 |
0.539 |
1.854 |
1.393 |
2.746 |
Halite |
-0.010 |
2.03 |
220 |
67.1 |
4.72 |
9.58 |
1.311 |
1.086 |
0.921 |
1.207 |
5.075 |
Gypsum |
0.490 |
2.35 |
172 |
52.4 |
4.04 |
9.49 |
1.093 |
0.408 |
2.451 |
2.678 |
3.232 |
Trona |
0.350 |
2.08 |
213.3 |
65.0 |
0.71 |
1.48 |
1.265 |
0.663 |
1.508 |
1.908 |
0.724 |
Sulphur |
0.020 |
2.02 |
400.3 |
122.0 |
5.05 |
10.20 |
0.728 |
1.065 |
0.939 |
0.684 |
5.489 |
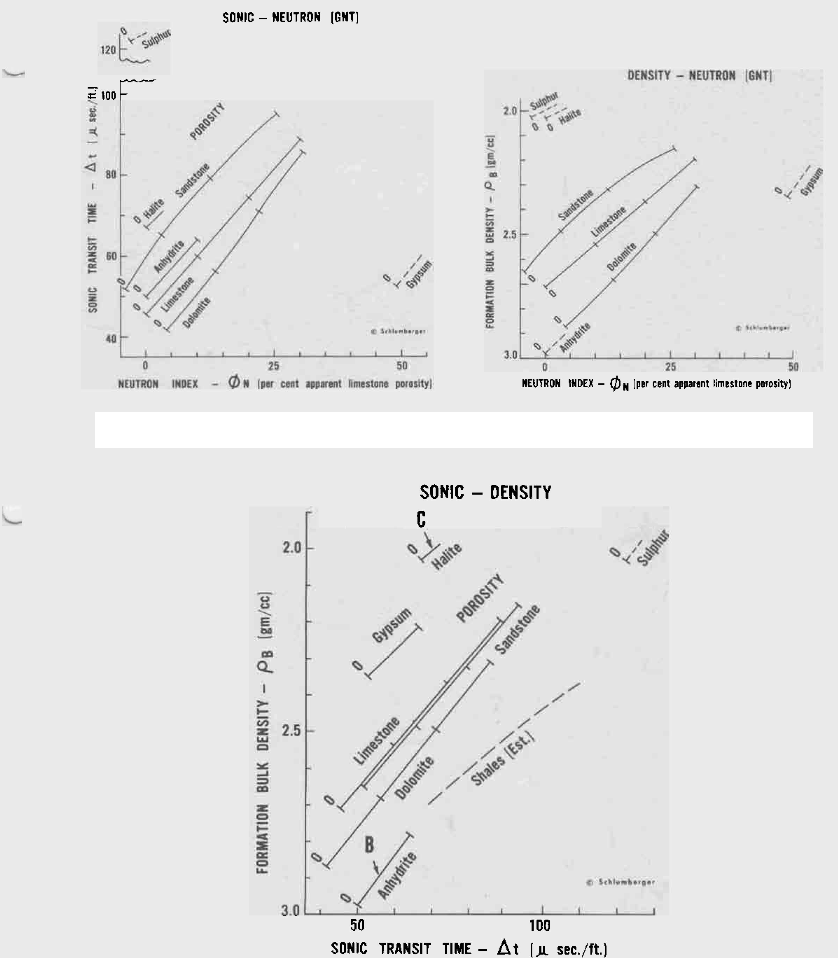
Standard crossplots with extended scales to show
positions of halite and sulphur relative to the common
sedimentary minerals.
References:
1. Defining Evaporite Deposits with Electrical Well Logs R. P. Alger, E. R. Crain,
P.Eng. Trans Northern Ohio Geological Society - Second
Symposium on Salt, Cleveland, 1966
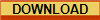
2. Log Evaluation of Non-Metallic Minerals
M.P. Tixier, R. P. Alger,SWSC, 1967, Geophysics Vol 35 #1, 1970
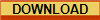
HALITE
with / without
ANHYDRITE
Halite or Rock Salt (NaCl) occurs in vast beds of
sedimentary evaporite minerals that result from the
drying up of enclosed lakes and oceans. Salt beds may be
hundreds of meters thick and underlie broad areas. In the
United States and Canada, extensive underground beds extend
from the Appalachian basin of western New York through parts
of Ontario and under much of the Michigan Basin. Other
deposits are in Ohio, Kansas, New Mexico, Nova Scotia,
Alberta, and Saskatchewan. Much of Europe into North Africa
and western Asia are underlain by salt beds. In most cases, other evaporites,
such as anhydrite (CaSO4)
and potash minerals, may be mixed or interbedded with the
salt.
Salt domes
are masses of salt that have been squeezed up from underlying salt
beds by the weight of overlying rock, coming closer to or breaching
the surface. Some domes are gypsum, not halite, but are still called
"salt domes" because gypsum is technically a salt, although it is
not very soluble and tastes terrible. Salt domes may contain
anhydrite, gypsum, and native sulphur, in addition to halite. They
are common along the Gulf coasts of Texas and Louisiana and are
often associated with petroleum deposits. Germany, Spain, the
Netherlands, Romania and Iran also have salt domes. Salt glaciers
exist in arid Iran where the salt has broken through the surface at
high elevation and flows downhill.
When exposed to water, anhydrite readily transforms to gypsum,
(CaSO4·2H2O) by the absorption of water. This transformation is
reversible, with gypsum forming anhydrite by heating to around 200 °C (400 °F)
under normal atmospheric conditions.
Halite is used to preserve and flavour food and is essential for
life. Industrial products are caustic soda
and chlorine. Salt is used in many industrial processes including
the manufacture of polyvinyl chloride, plastics, paper pulp, and many
other products. Of the annual global production of around 200 million tonnes,
about 6% is used for human consumption.
Salt can be derived from subsurface mining, solution mining, and
evaporation of seawater. Some mines produce a million tons a year or
more. Abandoned solution mines can be used for storage of natural
gas as they are virtually leak proof. Specialized radial acoustic
surveys are used to map the 3-D geometry of the cavern to assess its
volume and potential risks.
HALITE-ANHYDRITE EXAMPLE
Visual
Analysis rules:
Halite: gamma ray = near zero,
resistivity = very high, density = 2030 (2.03 g/cc), PE = 4.7, DTC =
200 (67 us/ft)), neutron = near 0.0
Anhydrite: gamma ray = near zero, resistivity = very high, density = 2970
(2.97 g/cc), PE = 5.0, DTC = 164 (50 us/ft)), neutron = near 0.0
These pure mineral values will vary by a small amount due to
occluded water and other mineral inclusions.
The following example illustrates the two common methods for
analyzing salt beds:
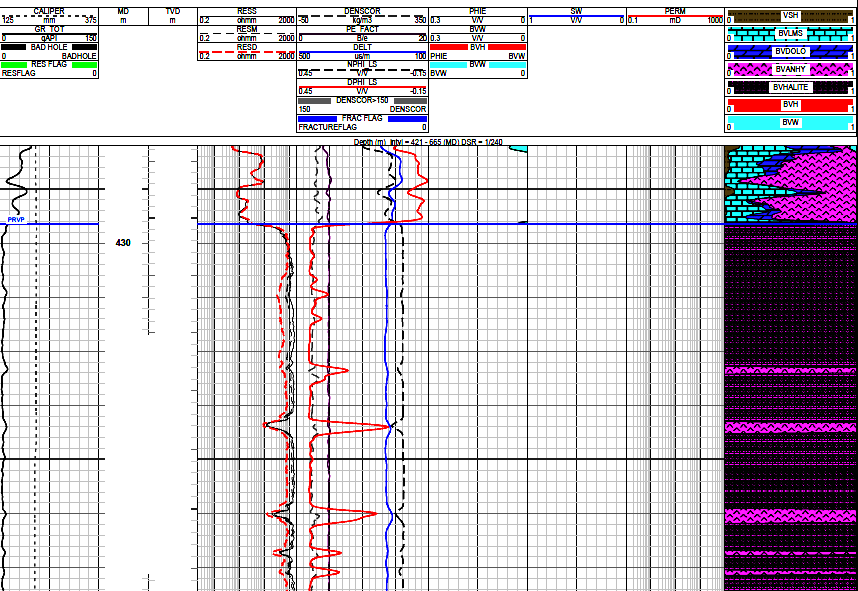
This example shows a very short portion of a log analysis of a salt
with interbedded anhydrite. The top 20 meters uses a calcite-dolomite-anhydrite
model with a PE-density-neutron 3-mineral model. A
halite-anhydrite 2-mineral model could have been used in the salt in
the lower portion of the image. However, results were determined
using lithology triggers instead, giving sharper definition to the
bed boundaries. Porosity in the salt is assumed to be zero so no
hydrocarbons are present. Note the low gamma ray, high resistivity,
and near zero neutron porosity. Density is near 3.0 g/cc in
anhydrite and near 2.0 g/cc in salt.
GYPSUM
Gypsum
(CaSO4·2H2O) is deposited from lake and sea
water, as well as in hot springs, from volcanic vapors, and
sulphate solutions in veins. It is often interbedded with the
minerals halite, anhydrite, and sulphur-bearing limestone.
When exposed to water, anhydrite readily transforms to
gypsum by the absorption of water.
When gypsum is heated in air it
loses water and converts first to calcium sulphate hemihydrate, (bassanite), often simply called "plaster" and,
if heated further, to anhydrous calcium sulphate (anhydrite).
It is used as a fertilizer, and as the main
constituent in many forms of plaster, blackboard chalk, and
wallboard. It is the hydrated form of anhydrite. A fine
grained version of gypsum is known as alabaster, used in
sculpture.
Gypsum is moderately water-soluble (2.0–2.5 g/l at 25 °C
compared to 360 g/l for halite) and, in contrast to most
other salts, it exhibits retrograde solubility, becoming
less soluble at higher temperatures.
A good example of an exposed gypsum deposit is White Sands,
New Mexico. A breached gypsum dome on Melville Island
(Barrow Dome) in the Canadian High Arctic was the subject of
a native sulphur exploration program in 1969. The sulphur
was limited to erosion remnants on the surface. The author
was project manager and a minor oil discovery was made at
shallow depths on the south side of the dome.
Gypsum is produced from open pit mines throughout Europe,
South Asia, Indonesia, Brazil, Canada, and USA. Some is
produced as a by-product of smoke stack scrubbers at coal
fired power plants.
GYPSUM EXAMPLE
Visual Analysis rules:
Halite: gamma ray = near zero,
resistivity = very high, density = 2030 (2.03 g/cc), PE =
4.7, DTC = 200 (67 us/ft), neutron = near 0.0
Anhydrite: gamma ray = near zero, resistivity = very high, density = 2970
(2.97 g/cc), PE = 5.0, DTC = 164 (50 us/ft)), neutron = near 0.0
Gypsum: gamma ray = near zero, resistivity = very
high, density = 2235 (2.35 g/cc), PE = 4.0, DTC = 173 (52
us/ft), neutron = 0.49
These pure mineral values will vary by a small amount due to
occluded water and other mineral inclusions.
Log
analysis parameters are somewhat similar to coal but PE of
coal is less than 1.0 and gypsum is near 4.0.
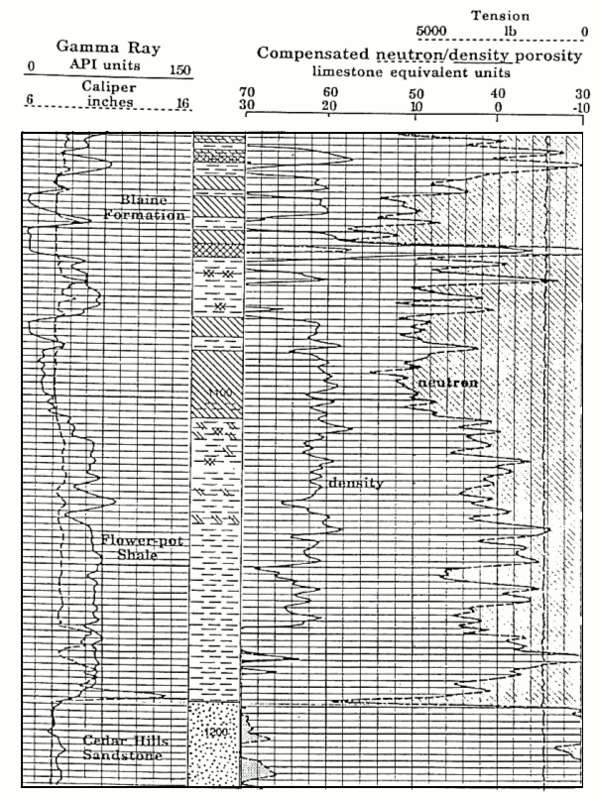
Gypsum example from western Kansas (image courtesy
KGS)
Porosity scale is -10 to 30 percent with backup from 40 to
70 percent. Gypsum is recognized by its low gamma -ray
value, very high neutron porosity exceeding 60% (due to the
hydrogen contained in its water of crystallization) and
density of 2.35 g/cc. The gypsum beds of the Blaine
Formation are obvious on the example log section, and can be
distinguished immediately from anhydrite, which has a
neutron porosity of near zero and a heavy density of 2.98.
An anhydrite bed is located at a depth of 1055 feet.
TRONA
Trona (Na2CO3•NaHCO3•2H2O) is an evaporite mineral formed in
seasonal lakes, now buried under more recent sediments, some
as deep as 1500 meters. Trona is found at Owens Lake and
Searles Lake in California, the Green River Formation of
Wyoming and Utah, the Makgadikgadi Pans in Botswana,
and in the Nile Valley in Egypt. Kenya, Turkey, and China
are also home to significant trona resources. Most trona is
recovered by subsurface mining, but small amounts are
produced from brine wells, and by
solution mining.
A related
carbonate
mineral,
nahcolite, has the composition of
sodium bicarbonate (NaHCO3.
It is mined in conjunction with or separately from Trona in
some areas.
Trona is the primary source of sodium carbonate (Na2CO3) in
the United States and to a lesser extent elsewhere. Sodium
carbonate is also known as "soda ash" and is rare in
nature. It has many domestic and industrial uses, such as
manufacturing glass, chemicals, paper, detergents, and
textiles. It is also used to condition water, remove sulphur
from both flue gases and lignite coals, and as a food
additive.
When trona is heated to 500'C, both water and CO2 are driven
off. This is followed by recrystalization from aqueous
solution leaving sodium carbonate suitable for delivery to
industrial customers. The USA has a vast reserve of trona,
so it uses the heating of trona to produce about 25% of the
world's supply. The alternate method of production is known
as the Solvay process. It produces sodium carbonate in large
quantities from halite (sodium chloride) and limestone
(calcium carbonate). China produces nearly 50% of the
world's supply, using both methods.
Many trona deposits are composed of multiple thin beds
interspersed with marl or clay. Logs may have difficulty
resolving beds less than 1 to 2 meters thick.
TRONA EXAMPLE
Visual Analysis rules:
Marl/Clay: gamma ray = 50 -100, resistivity =
low, density = 2300+/-
(2.30 g/cc), PE = 3.5, DTC = 328 (100 us/ft), neutron = 0.30 - 0.40
Trona: gamma ray = near zero, resistivity =
high, density = 2080 (2.08 g/cc), PE =
0.7, DTC = 213 (65
us/ft), neutron = 0.35
These pure mineral values will vary by a small amount due to
occluded water and other mineral inclusions.
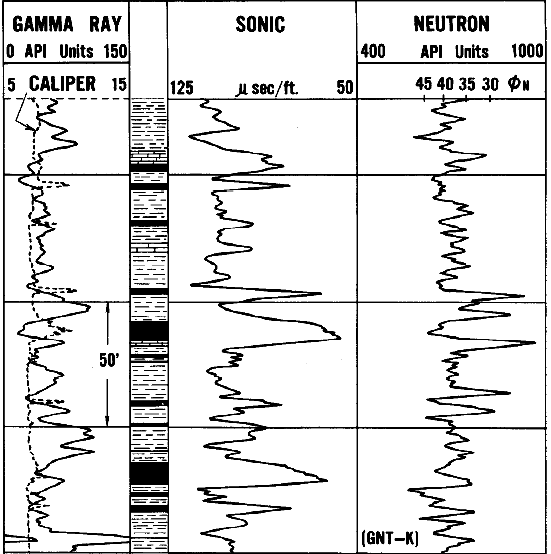
An ancient log from the Green River formation. Wyoming.
contains beds of trona. The properties of this mineral are
sufficiently different from the surrounding marl formation
that logs clearly locate the trona beds. The beds of
trona are indicated by black shading. Marl is indicated by
high GR and high sonic travel time, trona by low GR and
lower sonic. Neutron for both is near 0.35 to 0.40, helping
to eliminate other possible minerals in the clean intervals.
Modern density neutron and high resolution resistivity logs
would materially improve the visual interpretation.
Sulphur
The element Sulphur or
Sulfur is a non-metallic element and, like halite, it is
essential for life. In its native form, sulphur is a yellow
crystalline solid. In sedimentary rocks, it is synthesized by
anaerobic bacteria acting on sulphate minerals such as gypsum in salt
domes. Significant deposits in salt domes occur along the Gulf of
Mexico, and in evaporates in eastern Europe and western Asia. Salt
domes are associated with traps for oil accumulation, so in
drilling for oil considerable sulphur was discovered in the
same wells.
In salt domes, the sulphur is usually a physical mixture with
limestone, forming up to 50+% of the bulk volume of the
rock. Some water filled porosity may be present. More
complex mineral mixtures may also complicate the scene.
Elemental sulphur can also be found near hot springs and volcanic
regions in many parts of the world, especially along the
Pacific Ring of Fire; such volcanic deposits are currently
mined in Indonesia, Chile, and Japan.
Salt-dome sulphur was mined
by the Frasch process, in which superheated water was pumped into a
native sulphur deposit to melt the sulphur, and then compressed air
returned the 99.5% pure melted product to the surface. The melting
point of sulphur is 115'C so considerable energy was expended in
heating the sulphur zone. Throughout the 20th century this procedure
produced elemental sulphur that required no further purification. Due
to a limited number of such sulphur deposits and the high cost of
working them, this process for mining sulphur has not been employed
in a major way anywhere in the world since 2002.
Today, sulphur is produced mostly from petroleum, natural gas, and
related fossil resources, from which it is obtained mainly as
hydrogen sulfide (H2S). Known as an organosulphur compound, it can be
upgraded to produce near-pure sulphur, resulting in the large
yellow blocks of sulphur seen beside gas processing plants.
The sulphur is shipped from here by truck or train to the
many industrial firms that use it.
Elemental sulphur is used mainly as a precursor to other chemicals.
Approximately 85% is converted to sulphuric acid (H2SO4). The
principal use for the acid is the extraction of phosphate ores for
the production of fertilizer manufacturing. Other applications
include oil refining, wastewater processing, and mineral extraction.
Sulphur itself is used in the manufacture of cellophane, rayon,
medicines, and insecticides, Sulphates are used as a bleach for
paper, as a component of Portland cement, and as fertilizer.
If the use of fossil fuels is reduved
over the next several decades, as government mandates
insist, sulphur from this source will also decrease – we may
end up using the Frash process again or maybe there ia a
cheap way to pull sulphur out of gypsum (CaSO4.2H2O).
Petrophysical analysis of logs for sulphur exploitation is no longer
common, but we still need to be able to recognize it. Sulphur looks a
lot like
porosity on sonic and density logs, and with the high resistivity,
it can be mistaken for a hydrocarbon zone based on standard
visual analysis rules. There are some rules below that might help avoid
this problem.
Quantitative methods reduce the risk of misinterpretation. Three-mineral simultaneous equation model with calcite,
sulphur, and water
will work, using sonic, density, and neutron data. If another
mineral is present, such as anhydite or gypsum, lithology triggers
or a fourth equation using the PE curve can be added.
By
using the characteristic mineral and water properties in the
response equations for the limestone-sulphur case, we get:
1: Vsulphur = (PHID - PHIN) / 0.40
2: Vsulphur = (PHIS - PHIN) / 0.53
Where:
PHID = density porosity on a limestone scale (fractional
PHIN = neutron porosity on a limestone scale (fractional
PHIS = sonic porosity on a limestone scale (fractional
SULPHUR EXAMPLE
The above equations lead to some simple visual rules for a calcite-sulphur mixture:
1. PHIN = actual water filled porosity
2. PHID = PHIN = Limestone, with no sulphur, water or oil depending on
resistivity
3. PHID > PHIN = limestone, with sulphur
4. PHID << PHIN = Anhydrite, no porosity, no sulphur
Ancient density
neutron overlay in sulphur bearing rock. Diagonal
crosshatch = sulphur zone, bricks = limestone, xxxx = anhydrite ==>.
|
|