Metallic minerals
This article reviews conventional borehole logs that are
useful in the mining sector, followed by a more detailed
discussion of some less familiar special-purpose logs with
specific application to metallic mineral exploration. Both
have a significant part to play in the evolving quest for
greener energy solutions, in finding commodities, and
solving environmental issues.
There is much common ground in the geoscience of mineral and
petroleum exploration and development, but also some
fundamental differences. For example, mining operators rely
on drill-cores more than borehole logs. Mine integrity and
the safety of underground workers is part of the reason.
Also pertinent is that miners need to know the mineral
composition of an ore body with more precision than logging
tools could offer. Regardless, logs and cores are used in
both industries, so we will explain the differences and
overlaps in these two communities.
Decarbonizing by electrifying the World is a monumental
task; a variety of reports claim that metallic mineral
outputs may not keep pace with demand for power grid
upgrades or water and wind turbine construction, let alone
all those electric vehicles. Deeper new mines and expansion
of older mines are urgently needed; leading to increased
demand for geoscientists and engineers capable of locating,
detailing, and operating these new mines. Failure to match
mineral supply to expected demand will mean failure of any
plan for a “net-zero” economy.
Hopefully, the reader will be inspired to consider new,
cooperative ways to improve our mineral outputs and meet the
tough challenges ahead.
Location of Mineral Deposits in Canada, including Rare Earth
Elements
borehole logging IN the Mining ENVIRONMENT
For
clarity, we will refer to logs run for the mining industry as
“borehole logs” and those for the oil and gas industry as “oilfield
logs” or “well logs”, even though the guiding physical principles
are the same for both.
It is difficult to make direct comparisons between oilfield logging
tools and borehole tools. Many contractors developed their own
tools and probes are often customized to suit a particular
exploration challenge. The result is less standardization. Some
contractors offer a complete range of services from data acquisition
to mapping, while others specialize in smaller projects, by
supplying tool rentals. Happily, many of the borehole log names are
well-known to the oilfield log analyst, as the measurement
principles are the same. Acoustic, gamma ray, spectral gamma ray,
density, neutron and electrical logs are common to both industries.
In general, borehole tools are smaller and have reduced temperature
and pressure ratings (eg., 20 mPa and 80 degC) compared to oilfield
tools (100 mPa and 150 degC). However, many standard oilfield tools
are available in slim-hole versions and are quite suitable for
mineral borehole logging. A typical slim-hole gamma ray tool is just
42.9 mm (1-13/16 in) in diameter and approximately a meter long,
compared to a mineral service contractor’s GR tool at 38 mm diameter
and length of 0.63 meters.
There is a striking difference in scale between borehole logging
operations for mining, and that for petroleum. Mining drill-rigs
are typically portable (even heli-portable), and boreholes are
drilled to recover core or, in the case of reverse circulation (RC)
drilling, to recover samples. Boreholes can be blasted or drilled,
with logging equipment typically consisting of 3 components: a data
acquisition system to collect data from the downhole probe, a winch
to deploy the probe into the borehole, and the downhole probe
itself, which might be standalone or stackable.
borehole logging and coring Programs
The primary
logging measurements would be one or more of the following:
electrical conductivity (or resistivity), magnetic susceptibility,
natural gamma radiation (total and spectral), acoustic velocity (or
travel time), bulk density, and more recently, induced gamma ray
spectroscopy to identify particular metallic elements in the host
rock.
Specialty logs such as magnetic susceptibility, induced
polarization, or high resolution temperature logs may be used as
well.
Terraplus in Canada, offers auxiliary equipment such as video
inspection systems, borehole geophones, and hydrophone arrays, plus
ground penetrating radar antennas for single hole investigation and
cross-hole tomography. In the USA, Century Geophysical, among
others, provides a wide variety of tools for the mining industry.
The service providers are usually local contractors or the mining
company itself.
Geological Survey of
Canada and the US Geological Survey have
also developed their own logging tools, mostly used in mineral
reconnaissance surveys.
The mining
industry relies heavily on coring, core description, and lab work
for its geotechnical and geomechanical logs. Very detailed
lithology, stratigraphy, and structure are annotated on these logs,
as well as detailed notes on grain size, texture, and rock fabric.
This information is entered into 3-D modeling software. Rock
strength, discontinuities, faults, and fractures are carefully
mapped into the model. Borehole logs and core photos are added to
complete the 3-D display.
The model is constantly updated throughout the feasibility, design,
development, operational, and expansion phases of a mines long
lifetime. The integrity of the mine and the safety of the workers
depend on the accuracy of this model. No shortcuts allowed!
The coring and logging procedures described above are also used to
study geomechanical properties for dams, tunnels, highways,
foundations, and many other large construction projects.
SPECIALIZED Logging TOOLs FOR MINING APPLICATIONS
This
section describes some of the specialized borehole logging tools
used in mineral exploration and development, including some new or
experimental tools that may solve some problems that conventional
tools cannot. With the exception of induced gamma ray spectroscopy
and limited use of induced polarization, these tools are not used in
oilfield situations.
INDUCTION / Electromagnetic Susceptibility Logs
Electromagnetic methods are familiar to the oil and gas
sector as induction logs. They were developed due to the popularity
of oil-based mud and air-drilling systems. There was early
recognition that having control data from a borehole instrument
would verify the interpretation of surface magnetometer studies.
(5)
In the mining sector, a probe consisting of a transmitter and
receiver induces current flow in the formation creating a primary
magnetic field. This causes eddy currents to flow in a continuous
circular distribution centered around the borehole axis. These eddy
currents are proportional to the formation conductivity, and they in
turn generate a secondary magnetic field, which induces an
alternating voltage in the receiver coil. In the resulting voltage
vector, the magnitude and phase are a function of the conductivity
of the formation. Phase
sensitive detectors separate the signal into its resistive (from
conductivity) and reactive (from magnetism) components so that the
electrical conductivity and magnetic susceptibility are recorded
independently and simultaneously.
The log displays magnetic susceptibility, (c
(chi)),
either in cgs^106 or SI units, and conductivity in mmho/m
(mS/m). Chi
relates a material’s magnetization M, to the strength of an applied
magnetic field H, using the equation M = Chi * H.
Simplified diagram of Electromagnetic tool showing phase shift
between conductivity and susceptibility.
(5)
Magnetic
anomalies may be caused by primary igneous or sedimentary processes,
or by secondary alterations where magnetic material is either added
or removed. Conductivity measurements can be used to detect and
correlate conductive sulphides. Magnetic susceptibility can directly
detect iron ore and be used to characterize and correlate sulphide
units. Main benefits of borehole electromagnetic methods are their
indifference to borehole rugosity and their ability to make
measurements through PVC casing.
Values of Magnetic Susceptibility in various formations, in cgs
^ 106 units.
(5)
In 2011,
the Geological Survey of Canada sponsored development of a new
triple sensor probe (TSP), which combined an electromagnetic
component to measure magnetic susceptibility, alongside a capacitive
resistivity sensor and high-resolution temperature device. The TSP
allowed three measurements to be taken concurrently in a single
logging run. The resistivity component was re-designed to use a
capacitor array formed from parallel rods instead of galvanic
contact electrodes which are useless in PVC cased or air-filled
holes. An oscillator drives the capacitor array to produce the
electrical field. This tool combination has found success in
delineating base metals such as Cu-Pb-Zn massive sulphides and
nickel-sulphide deposits. (6)
Figure
4: Diagram of Capacitor Array for TSP logging tool
(6)
Results from a TSP log showing a lithology track, sulphide
occurrences, resistivity, susceptibility and temperature.
(6)
Induced Polarization Logs
Induced
polarization is a method to image the conductivity and chargeability
of porous rocks. It is most commonly used to delineate disseminated
sulphides within a host rock. When a charging current is turned off,
voltage decays over a finite time (discharging) back to zero. When
the current is turned on, voltage builds up over a finite time to a
maximum applied value. The current is for a time stored in the
ground (capacitance), causing some material to become polarized.
This phenomenon is called induced polarization. (7)
Century
Geophysical has a multi-parameter Series Induced Polarization
logging tool for hard rock mining and uranium exploration. It
records SP, a single point resistance, and induced polarization,
with optional natural gamma ray. The tool features a 16 and 64
array electrode spacing, passing an alternating current through the
formation, resulting in a measurement of chargeability. This
lightweight tool is 51 mm. OD and ~2 m. long.
Material Type
1 % Sample Concentration |
Chargeability (msec) |
Pyrite |
13.4 |
Chalcocite |
13.2 |
Copper |
12.3 |
Graphite |
11.2 |
Chalcopyrite |
9.4 |
Galena |
3.7 |
Magnetite |
2.2 |
Malachite |
0.2 |
Hematite |
0 |
Chargeability times for various rocks and minerals.
(7)
In 1986, a
Canadian study ran IP logs along with temperature, resistivity, and
SP logs to evaluate IP’s usefulness in finding gold associated with
pyrite within a volcanic host rock. The combined logs were very
good at defining a marker bed of graphite-schist but proved more
ambiguous for the gold. The study showed the benefits of
multiparameter logging acquisitions for delineating highly altered
rocks, but a statistical approach to the
complex dataset needed further development. (8)
Comparison of Gold Assay, track 1 with IP response, track 5.
(8)
Induced Gamma Ray Spectroscopy
Induced
gamma ray spectroscopy logs, sometimes called activation logs,
measure the concentration of specific elements in the rock. In
oilfield use, the elemental yields are transformed into minerals
using a least squares algorithm to create a lithology log
presentation. Capture cross section (Sigma) and neutron porosity
(TPHI) are the other primary measurements, carried forward from the
earliest days of the pulsed neutron log. These two measurements
allow us to calculate porosity and water saturation in cased holes.
In mining
exploration, this technology has lagged behind resistivity and gamma
ray methods, mainly due to mining’s reluctance to handle radioactive
sources in the field. This is not surprising when the logging unit
could be the back of a geologist’s truck, plus developing such a
specialized tool could be prohibitively expensive.
Pulsed neutron methods were being used in mining in 1972, initially
to detect copper. The development of a delayed-fission neutron
system, by Century Geophysical Corp. was found to be superior for
uranium prospecting. (9)
More
recently, CSIRO in Australia has developed a Prompt Gamma Neutron
Activation Analysis (PGNAA) (10), a spectrometric nuclear
logging tool which results in a real-time downhole assay of elements
in the rock. Similar in principle to the LithoScanner and
Pulsar tools from the petroleum industry, the PGNAA bombards the
formation with fast neutrons and measures the gamma ray spectra
generated by the nuclear reaction. The gamma rays’ intensity and
energy create unique photopeak signatures, proportional to the
elemental composition of the rock. The tool samples the surrounding
rock to a depth of ~50 cm, generating in situ rock mass density and
estimates of elemental composition. A main advantage over the core
assay method is continuous depth coverage (no lost core!) plus
deeper depth of investigation. The tool is calibrated to the
expected mix of mineralogy and source/detector configuration is
designed specifically for that expected lithology.
The PGNAA
tool may feature either chemical or pulsed neutron sources and uses
either BGO (NaI(T1) or CsI
detectors.
The measurement range is slightly larger than oilfield tools at 0.5
to 11 MeV. The tool has several shields, to protect the detector
from fast and thermal neutrons escaping from the sample, and from
the primary gamma rays generated by the source. A biological shield
is built-in to minimize radiation risk to logging personnel.
The tool
quantifies elements such as Si, Fe in iron ore, Mn in manganese ore,
Cu, Ni, Ti, Cl, and many more. A benefit is that the large range of
discoverable elements makes it possible to quantify “penalty”
elements, which lower the grade of ore, cause smelting problems, or
introduce unwanted attributes in the finished product, such as
brittleness to steel.
In contrast, oilfield tools such as Schlumberger’s open-hole
LithoScanner or cased-hole Pulsar use a pulsed neutron generator.
LithoScanner focuses on the element sets common to petroleum
environments (silicates, carbonates, unconventional), with just 4
metals of interest to mining: Cu, Gd, Ni and Ti. Although the
PGNAA’s element sets are geared to hard rock environments, the tools
have 15 elemental outputs in common
The Pulsar log is a slim-hole 42.7 mm (1-11/16 in), 5.5 m long
alternative to LithoScanner. Pulsar provides a similar suite of
elemental logs (with additional elements on request) and an accurate
measure of TOC. Its main advantage is the fast neutron cross
section (FNXS), a measurement that, being independent from
resistivity-based calculations, is a fool proof gas indicator. The
results are very helpful in monitoring CO2 storage, CO2 miscible
floods, and helium reservoirs. In mining boreholes, it may provide
information suitable for correlation and quantification of massive
sulphides.
Comparison of laboratory assays with SirologTM PGNAA
values for lead.
(10)
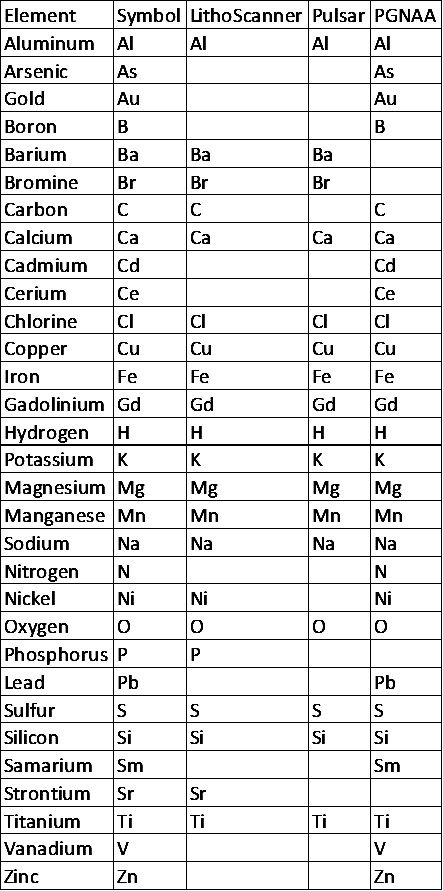
Comparison of elements “seen” by various tools.
High Resolution Temperature Logs
In the
1980s, the Borehole Geophysics Group of the Geological Survey of
Canada developed a sensor to quantify borehole temperature to a
resolution of 0.001 degree Celsius. (6) Roke Oil
Enterprises in Calgary developed a tool with the same resolution
about the same time and offered the service commercially. The
resolution of most tools is 0.01C.
Temperature gradients can change by formation and results can be
used to map thermal conductivity contrasts, plus detect massive
sulphides. Other applications include predicting proximity to old
mine workings, where the heat dissipates from the warmer underground
openings, finding the base of
permafrost, understanding groundwater flow patterns, and locating
gas flows through “worm-holes” in the cement behind casing.
|