INELASTIC AND
CAPTURE SPECTROSCOPY BASICS
Activation, or induced gamma ray
spectroscopy,
logs record concentrations of individual chemical elements derived
from the characteristic energy levels of gamma rays emitted by a
nucleus that has been activated by neutron bombardment. Pulsed
neutron spectroscopy and elemental capture spectroscopy are other
common names for this kind of log. Chlorine, oxygen activation,
aluminum activation, and carbon oxygen logs also fall into this
category, as well as the reservoir saturation tool (RST), the
Litho-Scanner, and Pulsar logs.
The geochemical log (GST) and its successor the elemental capture
spectroscopy log (ECS) were widely used for hydrocarbon and mineral
identification. The GST and ECS could be run in either cased or open
hole. Slow logging speed reduced their early acceptance by industry
but the modern versions of spectroscopy logs are run at
normal speeds due to much improved detector design.
Historically, cased hole
tools analyzed carbon/oxygen ratios from the high-energy
neutron inelastic scattering spectra. In contrast, open
hole tools were initially constructed to evaluate lower
energy nuclear capture interactions. In fact, the tools
could be run in either open or cased holes.
The
tools utilize one of two source types: chemical refers to an AmBe
source with an average neutron output of 4.2 MeV (ECS), or pulsed
neutron generator (PNG), which bombards the formation at 14.1 MeV.
The newest versions, LithoScanner and Pulsar (Schlumberger) deliver
near simultaneous data gathering from both inelastic and capture
spectra, meaning better inputs for petrophysical interpretation.
These tools, their predecessors and successors are described
here in chronological order so you can see the evolution of
tool capability and to help you assess the value of the data
in your well files.
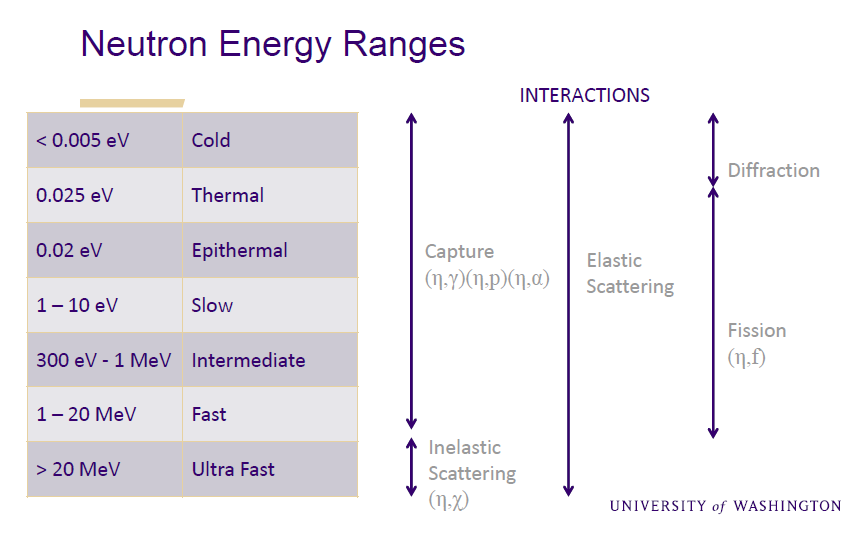
Chemical
sources emit neutrons in the slow range; PNG tools emit energy in
the fast range.
CHLORINE LOG (CLT)
The first
incarnation of an elemental capture spectroscopy log was the
chlorine log. The recorded curve was a measure of the concentration
of chlorine in the formation. High chlorine meant salt water in high
to moderate porosity. Low chlorine meant hydrocarbon or fresh water
or low porosity. By using the porosity log, we could sort out low
porosity but sorting hydrocarbon from fresh water required local
knowledge. This tool was rare, usually run through casing but open
hole examples exist.
CARBON OXYGEN LOG (C/O)
The next incarnation of an elemental
capture spectroscopy log was the carbon/oxygen log. It presented
a log curve of carbon/oxygen ratio, a fraction (C/O) and
silicon/calcium ratio (Si/Ca). High C/O indicate
hydrocarbons as opposed to water and high Si meant sandstone as
opposed to carbonate rocks. Shale and mudstone should have low
C/O and low Si/Ca, except silty shale could have moderate Si/Ca
ratios. Count rates were measured in counts per minute instead
of counts per second, so the tool had to be run very slowly as
a through casing tool. It worked best in high porosity. A C/O
log is incorporated in most recent versions of induced
spectroscopy tools. The principle behind the C/O log is
inelastic neutron scattering.
PULSED NEUTRON SPECTROSCOPY LOG
Pulsed neutron spectroscopy log
is a wireline log of the yields of different elements in the
formation; measured using induced gamma ray spectroscopy with a
pulsed neutron generator.
This log should not be confused with
pulsed neutron or thermal decay time (TDT) logs, which
measure quite different rock properties.
While chemical source tools emit
slow neutrons, meaning only elements from the capture spectrum
are counted, the PNG's elemental yields are derived from
two intermediate results: the inelastic and the capture
spectrum. The inelastic spectrum is the basis for the
carbon-oxygen log, and can also give information on other
elements. The capture spectrum depends on many elements, mainly
hydrogen, silicon, calcium, iron, sulfur and chlorine.
Since the elemental yields give information only on the relative
concentration of elements, they are normally given as ratios,
such as C/O, Cl/H, Si/(Si + Ca), H/(Si + Ca) and Fe/(Si + Ca).
These ratios are indicators of oil, salinity, lithology,
porosity and clay, respectively. The main purpose of the log is
to determine lithology, the principal outputs are the relative
yields of silicon, calcium, iron, sulfur, titanium and
gadolinium. The yields give information only on the relative
concentration of these elements. To get absolute elemental
concentrations, it is necessary to calibrate to cores, or, more
often, use a model such as the oxide-closure model.
The depth of investigation of the log
is several inches into the formation. It can be run in open or cased
hole. Pulsed neutron spectroscopy logs were introduced in the mid
1970s after a decade or more of investigation. Early tools were
physically long, expensive and required complex interpretation
models.
INDUCED GAMMA RAY SPECTROSCOPY LOG aka
GEOCHEMICAL LOG (GST)
The
induced gamma ray spectroscopy log, also called the geochemical log, is a more recent
spectroscopy incarnation and was run in
cased hole as the GST tool and in open hole as the GLT tool
(Schlumberger terminology). It is a log of elemental
concentrations from which the geochemistry of the formation may
be derived.
Raw log curves for a GST log
The words "geochemical log" as used here should not be confused
with the same term used to describe laboratory procedures to
determine quite different
chemical properties of rocks.
Several different logs provide information on
elemental weight concentrations: natural gamma ray spectroscopy,
elemental capture spectroscopy or pulsed neutron spectroscopy,
and aluminum activation. The combination of all of their outputs
is known as a geochemical log, since it provides information on
most of the principal elements found in sedimentary rocks.
As for the
pulsed neutron spectroscopy log, absolute concentrations can be
derived by calibration to core or by using a model such as the
oxide-closure model. The absolute elemental concentrations can
then be converted into mineral concentrations using a model that
defines what minerals are present. The first complete
geochemical logs were run in the mid 1980s.
The
oxide-closure model for converting relative elemental yields from a
pulsed neutron spectroscopy log to absolute weight concentrations
uses the assumption that the sum of all oxides in the rock matrix is
1.00. The model is based on the observation that, with few
exceptions, sedimentary minerals are oxides, so that the sum of the
dry weight percent of all oxides must be 100%. The weight percent of
an oxide can be calculated from the dry weight percent of the cation
by knowing the chemical formula.
The absolute dry
weight fraction, W, of element i is given by:
1: Wi = F * Yi / Si
Where:
F = unknown normalization factor
Yi = measured spectral gamma ray yield
Si = tool sensitivity to that element, measured in the laboratory.
The dry weight
fraction of the oxide is then:
2:Oi = F * Xi * Yi / Si
Where:
Oi = the oxide association factor, given by the chemical formula.
Since the sum of all Oi equals 1.00, it is possible to calculate F
and determine each Wi .
ELEMENTAL CAPTURE SPECTROSCOPY LOG (ECS)
The elemental capture spectroscopy (ECS)
log was the next version of activation logging. Unlike earlier
versions it does not use a pulsed neutron source but uses
instead a standard
americium beryllium (AmBe) neutron source
and a large bismuth germinate (BGO) detector to measure relative
elemental yields based on neutron-induced capture gamma ray
spectroscopy. The primary elements measured in both open and
cased holes are for the formation elements silicon (Si), iron
(Fe), calcium (Ca), sulfur (S), titanium (Ti), gadolinium (Gd),
chlorine (Cl), barium (Ba), and hydrogen (H).
Wellsite
processing uses the 254-channel gamma ray energy spectrum to
produce dry-weight elements, lithology, and matrix properties.
The first step involves spectral deconvolution of the composite
gamma ray energy spectrum by using a set of elemental standards
to produce relative elemental yields. The relative yields are
then converted to dry-weight elemental
concentration logs for the elements Si, Fe, Ca, S, Ti, and Gd
using the oxides closure method. Matrix properties and
quantitative dry-weight lithologies are then calculated from the
dry-weight elemental fractions using empirical
relationships derived from an extensive core chemistry and
mineralogy database. The ECS tool was a mere 10.2 feet
long, beginning the trend to shorter, slimmer tools with an
increased logging speed, making them more attractive to
industry.
The outputs are dry-weight lithology fractions
(from elements)
■ total clay
■ total carbonate
■ anhydrite + gypsum from S and Ca
■ QFM (quartz + feldspar + mica)
■ pyrite
■ siderite
■ coal
■ salt
Matrix properties (from elements)
■ matrix grain density
■ matrix thermal and epithermal neutron
■ matrix sigma.
Applications
■ Integrated petrophysical analysis
■ Clay fraction independent of gamma ray, spontaneous potential, and
density neutron
■ Carbonate, gypsum or anhydrite, pyrite, siderite, coal, and salt
fractions for complex reservoir
analysis
■ Matrix density and matrix neutron values for more accurate porosity
calculation
■ Sigma matrix for cased and open hole sigma saturation analysis
■ Mineralogy-based permeability estimates
■ Quantitative lithology for rock properties modeling and pore pressure
prediction from seismic data
■ Geochemical stratigraphy (chemostratigraphy) for well-to-well
correlation
■ Enhanced completion and drilling fluid recommendations based on clay
versus carbonate cementation
■ Coalbed methane bed delineation, producibility, and in situ reserves
estimation
RESERVOIR SATURATION LOG (RST)
The reservoir saturation tool (RST) is
a combination of a modern carbon oxygen log and a standard
pulsed neutron log.
The dual-detector spectroscopy system of the
through-tubing reservoir saturation tool enables
the recording of carbon and oxygen and dual burst thermal decay
time measurements during the same trip in the well.
The
carbon/oxygen (C/O) ratio is used to determine the formation oil
saturation independent of the formation water salinity. This
calculation is particularly helpful if the water salinity is low
or unknown. If the salinity of the formation water is high, the
dual burst thermal decay time measurement is used. A combination
of both measurements can be used to detect and quantify the
presence of injection water of a different salinity from that of
the connate water.
Applications
■ Formation evaluation behind casing
■ Sigma, porosity, and carbon/oxygen measurement in one trip in the
wellbore
■ Water saturation evaluation in old wells where modern open hole logs
have not been run
■ Measurement of water velocity inside casing, irrespective of wellbore
angle (production logging)
■ Measurement of near-wellbore water velocity outside the casing
(remedial applications)
■ Formation oil volume from C/O ratio, independent of formation water
salinity
■ Flowing wells (in combination with an external borehole holdup sensor)
■ Capture yields (H, Cl, Ca, Si, Fe, S, Gd, and Mg)
■ Inelastic yields (C, O, Si, Ca, and Fe)
■ Three-phase borehole holdup
■ PVL* Phase Velocity Log
■ Borehole salinity
■ SpectroLith lithology indicators Nuclear
LITHO-SCANNER LOG
The current generation lithology
tool is called LithoScanner (Schlumberger terminology). ECS’s
chemical AmBe source was replaced with a high-output pulsed
neutron generator (PNG) and the BGO detector superseded by a
LaBr3:Ce scintillator having 3 times better resolution. These
design changes increase the neutron output by a factor of ~7
over a chemical source. Inelastic interactions are separated
from capture data due to source timing; a pulse-height
histogram, or spectra, is generated during and after each
neutron burst. Some elements, such as carbon and oxygen, have a
signature in just one spectra; others are present in both,
meaning some elements are measured with greater precision while
other elements are newly quantified. Twenty-one elements making
up eight mineral groups are output, including magnesium, which
allows the differentiation of dolomite from calcite, and
aluminum for computing clay volume. Inorganic carbon is measured
and subtracted from total inelastic carbon, giving a stand-alone
TOC. This is key to computing kerogen volume in shale gas
plays. Additionally, elements such as copper can be used for
metal ore assays.
Importantly,
unlike a chemical source tool, the Pulsed Neutron Generator does not
emit radiation unless activated, making it safer to use and more
environmentally friendly. The slim 4.5” OD tool has a depth of
investigation of 7 to 9” with a vertical resolution of 18” and a
logging speed up to 3600 ft/hour.
Outputs:
■
Elemental Yields
■
Elemental Weight
Fractions: Al, Ba, Br, C, Ca, Cl, Cu, Fe, Gd, H, K, Mg, Mn, Na, Ni,
O, P, S, Si, Sr, Ti
■
Total Organic Carbon (TOC)
■
Dry Weight
Mineral Concentrations – Anhydrite, Clay, Calcite, Coal, Dolomite,
Evaporite, Pyrite, QFM, Siderite
■
Matrix Properties
(Density, Neutron and Sigma)
Applications:
■
Shale Gas
Reservoirs (Kerogen Volume and Hydrocarbon Saturation from TOC)
■
Mechanical
Properties
■
Facies
Identification
■
Sequence
Stratigraphy and Clay Typing
PULSAR LOG
(PNX-A)
2015 saw the
introduction of a new measurement: FNXS, or Fast Neutron
Cross-Section, which detects neutrons induced from fast neutron
inelastic scattering. The Schlumberger tool, Pulsar, combines high
neutron outputs with multiple detectors in a 1.73” OD tool, designed
to be run in cased holes through tubing. The tool can be operated
rig-less and is a good choice for horizontals, or wells with
instability issues.
The major
application is the detection of gas zones: the fast neutron cross
section values are similar for both matrix and water but read much
lower in gas. This is presented in an FNXS TPHI curve overlay which
shows better resolution than the open hole density neutron gas
crossover method. Unique to cased-hole interpretation, it is no
longer necessary to have open hole density logs.
The tool logging
speed varies according to the acquisition mode, from 200 ft/hour in
inelastic capture mode, up to 3600 ft/hour in inelastic gas, sigma
and HI mode.
Outputs:
■
Sigma
■
HI Hydrogen Index
■
TPHI Thermal
Neutron Porosity
■
FNXS Fast Neutron
Cross-Section
■
Inelastic and
Capture Elemental Yields
■
Carbon/Oxygen
Ratio
■
Total Organic Carbon (TOC)
Applications:
■
Standalone
Formation Evaluation (bypassed zones, depleted reservoirs, wells
with no OH or ancient logs and Carbon Storage reservoirs)
■
Differentiate and
quantify gas zones from liquid-filled porosity or tight zones
■
Water saturation
regardless of salinity
■
Low-resistivity
pay evaluation
■
Well-to-well
correlation and sequence stratigraphy
■
Detection of
water entry and flow behind casing
EXAMPLE LOGS
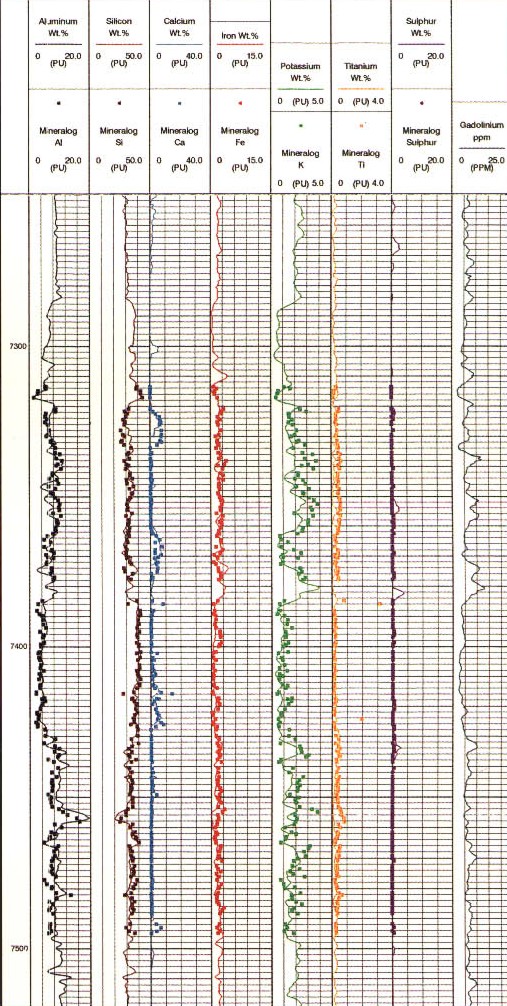
Weight percent curves from the oxide model for a GLT log.
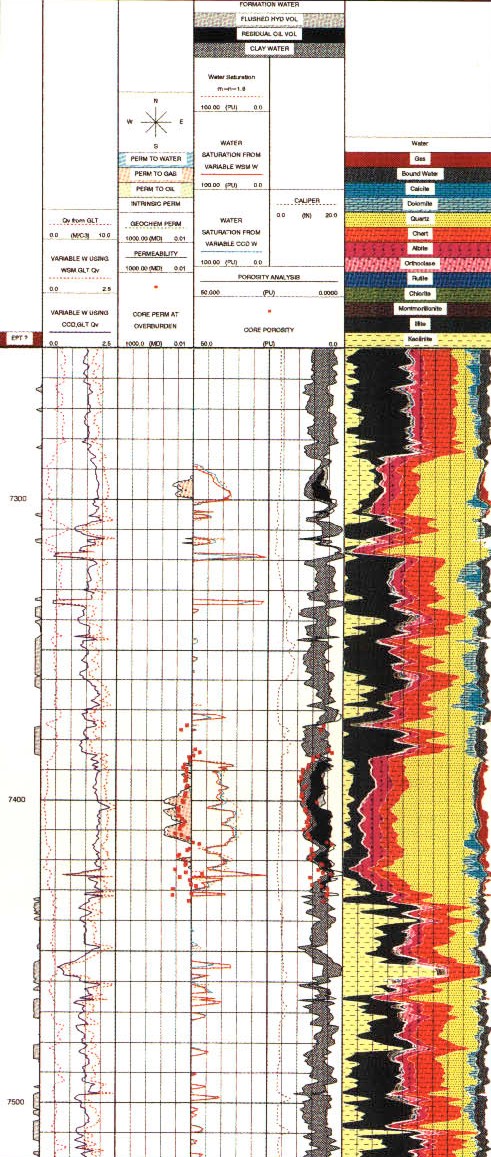
Computed lithology from oxide model, including porosity and
hydrocarbon saturation from C/O ratio.
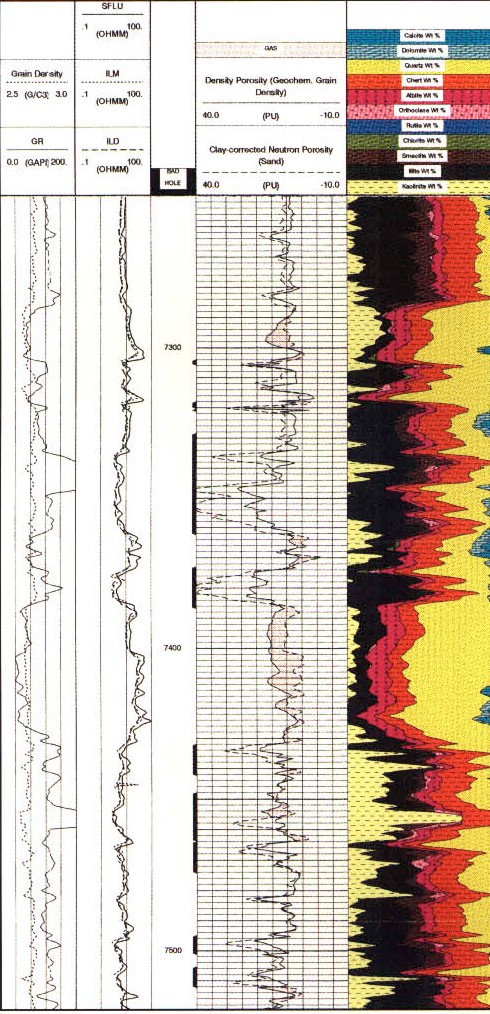
Alternate analysis for lithology and chromostratigraphy.
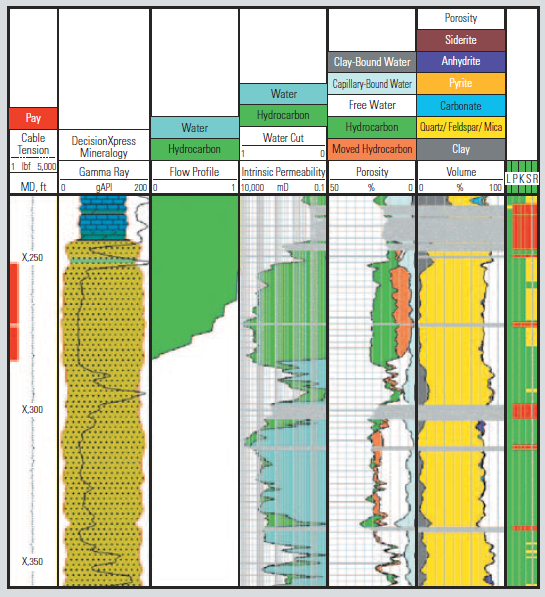
ECS Log showing volume track with mineral concentrations.
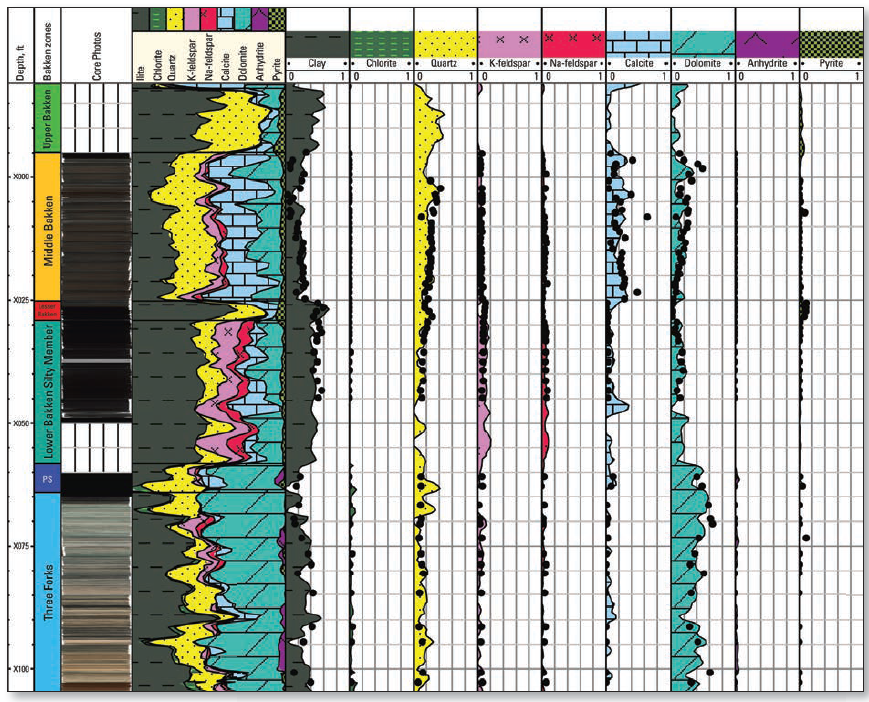
LithoScanner mineralogy showing increased elemental precision:
carbonates separated into calcite and dolomite; chlorite from
total clay.
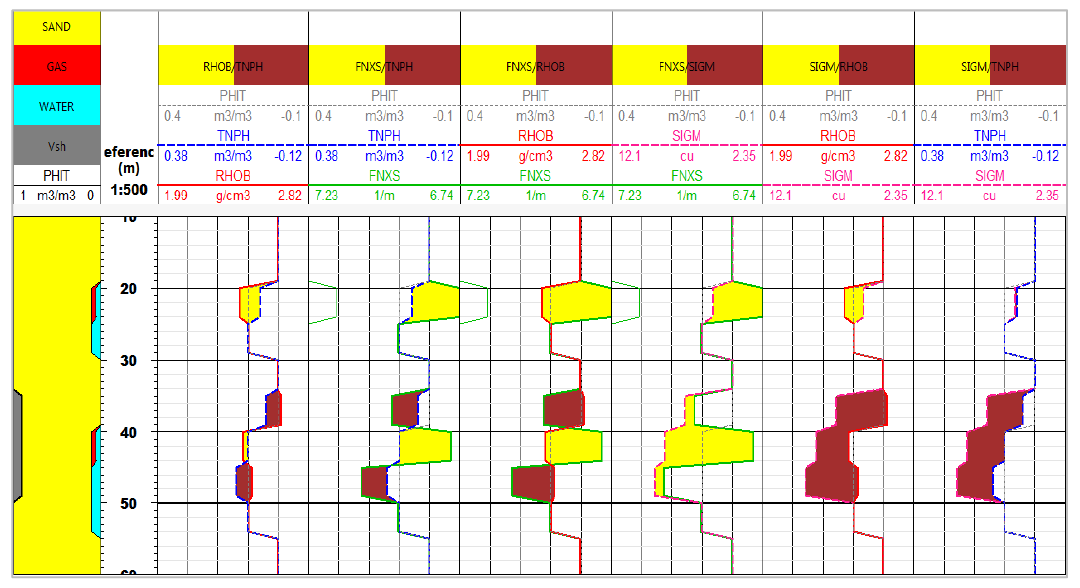
Pulsar Log: simulated log response modeled in sand/shale
sequence, comparing RHOB/TPHI with FNXS/TPHI overlays,
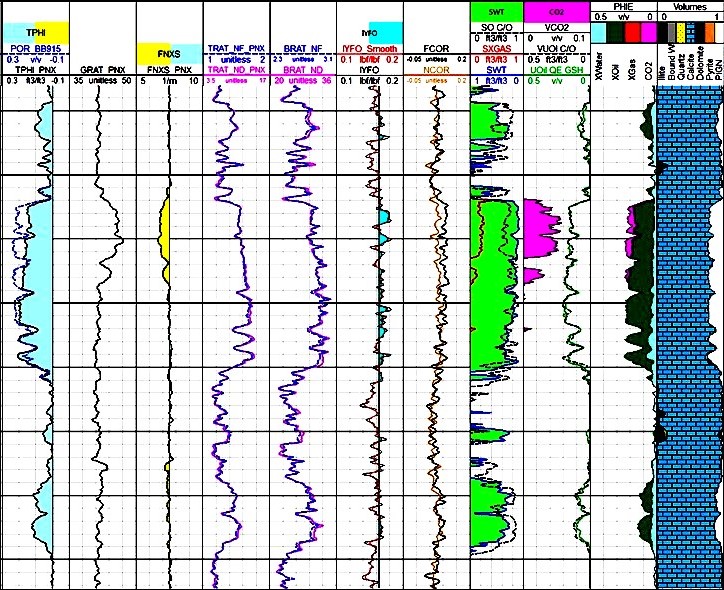
Examples of CO2 detection and quantification at current
reservoir condition. Different gas indicators are presented,
including Sigma, Neutron count rates and porosity, Fast
Neutron Cross Section, and its deviation from Fast Neutron
Cross Section of matrix components in presence of gas.
SIGMA, TPHI, FNXS end points calculated based on gas density
and composition. Lithology and
porosity are measured based on induced gamma ray
spectroscopy combined to TPHI and FNXS, eliminating the need
for open hole logs.
showing
better gas and clay response.
|