REPORT WRITING BASICS - KEEP IT SIMPLE
You will need blank forms or a computer and appropriate software
to
write your log analysis report. I currently use a spreadsheet
and word processor off the shelf, but corporate policy may force you to use more
cumbersome packages. The commentary should be written uniquely for each job, to
cover the who, why, what, when, where, results, and recommendations. Some “copy
and paste” is allowed but try to provide some original insights into each job.
Illustrative examples of crossplots, raw logs, answer plots, and
tables of results, for example net pay summaries, are commonly
included. Equations should be used only to explain a concept - the
complete computer code is not required. Copies of all depth plots
are usually delivered with the report as separate electronic images.
Tables are also delivered separately as electronic spreadsheets.
Tables
of results and graphs are usually
copied to the text document. These should be embedded near the
appropriate text, if possible. Large tables may appear at the end of
the report.
A
typical petrophysics report contains some or all of the following
topivs:
1.
Introduction or Executive Summary: this should include who the job
was done for, the overall objective of the project, names and
locations of wells and zones of interest, and a brief geological /
mineralogical description of the zones of interest.
2.
Data Available; describe log types and ages, core, XRD, petrography,
sample descriptions, perforation and test intervals, production
histories; comment on quality of each and especially what was
missing that could have been useful.
3.
Analysis Method; in words, explain the individual method used to
calculate shale volume, porosity, lithology, water resistivity,
water saturation, permeability, and net pay; describe how parameters
were selected, and how well log analysis results match core and lab
data; keep equations to a bare minimum. In unconventional
reservoirs, describe what factors about the reservoir are
unconventional and describe how additional parameters such as TOC
weight fraction and kerogen volume were calculated. If mechanical
properties were calculated, describe the log reconstruction method,
list the basic properties that were derived, and the presence of any
lab data that might be used for calibration.
4.
Discussion of Results; may be omitted if covered in methodology;
compare log analysis results to core and lab data, production
history, etc, on a well by well basis.
5.
Conclusions and Recommendations; discuss data quality, results
quality compared to core, missing data, further lab work needed;
come to a conclusion - the well (pool, project) is ......
6.
Disclaimer; you were not there, you didn't do it, and it's not your
fault anyway.
Your
name is on the report, be proud of it. Log analysis reports hang
around in well files for years. Don't leave a shoddy product that
will come back to haunt you.
Use
clear, positive statements. Write as if talking out loud to an
equal, but keep it organized and logical. Every sentence needs
at least one noun and one verb.
We all
learned to do this in high school physics lab, so it's not really
that hard.
Keep
it short and sweet - most petrophysical reports on small projects
are less than five pages of text plus cover page and tables of
results.
A full
field study may contain hundreds of pages from numerous authors, with geology, geophysics,
engineering, and simulation sections, 100's of maps and graphs and
well log displays. Keeping all this well organized and useful will
take some skill and effort. Short
reports don't need an executive summary but long reports definitely
do. Long reports also need a table of contents, table of
illustrations, and a clearly organized structure.
HOW
NOT TO
WRITE A REPORT
"Requisite
to a clear understanding of the interpretation of mud-gas
data is consideration of the source of hydrocarbons as
they occur in the drilling mud. To assist in this consideration,
a simple drilling model is proposed which illustrates
the impact of bit penetration through hydrocarbon accumulations.
A series of cases is presented where variations in the
configuration of the mud-gas data indicated specific differences
in the response of the hydrocarbon bearing zone to bit
penetration and subsequent rig operations.
The
model will show that the geometry of the gas kick recorded
by the instrumentation and plotted with respect to time
is directly related to significant characteristics of
the hydrocarbon zone as well as the impact of concurrent
drilling operations. It will become apparent that the
configuration of the gas kick as recorded directly from
the drilling mud is of greater interpretive significance
than the magnitude of the gas kick. When instrument chart
data recorded versus time is digitized and plotted in
graph format versus depth, the magnitude of the gas kick
may be faithfully reproduced but the configuration of
the kick is usually lost.
Thus
it becomes obvious that basic and vital interpretation
must derive from a detailed analysis of the instrument
charts themselves and not solely from a plotted graph.
The basic function of the plotted graph should be to collate,
according to depth, pertinent data produced from various
sources. This graph then provides a broader understanding
of the hydrocarbon accumulation and a convenient means
for future reference.
To
illustrate these concepts, a diagrammatic technique has
been employed which graphically relates the gas detector
response plotted versus time to the actual penetration
of the rock by the drilling bit through the penetration
rate curve plotted versus depth. This technique allows
direct comparison of the geometry of the gas kick to actual
rock penetration."
Scroll down to see four different, easy to read Petrophysical
Reports.
Petrophysical Analysis Report - Conventional
Reservoir
SAMPLE REPORT #1
24 Month 2012
Introduction
We were requested by Some One of Company A
to review the log, core, and test data on the subject well, and to
perform an independent petrophysical analysis on the A and T formations.
Available
Data
Log data for this project is relatively
sparse:
A Fm:
xxx
- xxx m No logs
xxx
- xxx m Induction, SP, Sonic, No GR or Caliper xxx
- xxx m As above plus GR, Caliper, poor quality
density
T Fm:
xxxx
- xxxx m As above
An
analyzed core was available just below the main porous interval
in the T Fm. Reported depths on this core appear to be 11 meters
shallow (approx one pipe joint). A second, deeper core was not
analyzed. No core was taken in the A Fm.
The
top of the T Fm was tested through perforations and produced some
wet gas. Eight separate intervals in the A Fm were tested through
perforations, indicating wet gas in the lower 50 meters.
No
Rw data was provided, so water saturation values from log analysis
are somewhat conjectural. No special core capillary pressure data
is available to help calibrate water saturation.
Analysis Method
Digital log curves for the well were provided by
the client. These were entered into Spectrum 2000 Mindware Ltd's
proprietary log analysis program called Meta/Log.
Shale
volume was determined from the gamma ray where possible and from
the resistivity log where GR was not recorded (250-550 m in A Fm).
The SP is quite flat and too smooth to be a useful shale indicator.
Porosity
was determined by the sonic log corrected for shale. The density
was also tried, but gave misleading results due to poor borehole
condition.
Water
saturation was derived with the Simandoux equation which corrects
for the effects of shale. An Rw equivalent to 85000 ppm NaCl was
used to achieve reasonable water saturations in the T Fm. A value
approximating 45000 ppm was used in the A Fm. There are no obvious
water zones, no RW data from offset wells, and no capillary pressure
data to calibrate water saturation results.
A generic
permeability curve using the Wyllie equation was generated but
not presented on depth plots, as core permeability is much lower
than the estimated values from this method.
Reasonable
cutoffs were chosen from experience in tight sands and hydrocarbon
summaries were printed. The zones that passed all cutoffs are flagged
on the depth plots.
Depth
plots at 1:1000 scale, brief summary listings, and this report
were FAXed to Some One on 24 Month 2012. Hardcopy with plots at
1:500 scale were delivered by courier.
Results
Results are contained in the depth plots and listings
supplied. Briefly these show:
Upper
T Fm: xxxx - xxxx mKB Phi = 0.093, Sw = 0.43, Net = 6.4 m
This zone was perforated and tested gas.
Middle
T Fm: xxxx - xxxx mKB Phi = 0.121, Sw = 0.27, Net = 6.4 m
This zone is not tested.
Lower
A Fm: xxx - xxx mKB Phi = 0.113, Sw = 0.51, Net = 50.4 m
Eight zones within this interval were perforated and tested some gas. Additional
intervals are untested and are flagged on the depth plots.
Upper
A Fm: xxx - xxx mKB Water saturation is speculative so no summations
have been run. Numerous resistivity bumps indicate cleaner sands
in thin intervals which might be gas bearing or they might contain
fresher water, analogous to the Belly River in Alberta.
Conclusions
There are many unknowns and assumptions in this log analysis,
more than in a typical project. Lack of RW data and special core data to calibrate
water saturation in any zone is a severe handicap. Results are based on personal
experience and the production tests.
The
lack of adequate density and neutron log data prevents the calculation
of porosity corrected for heavy minerals. Since volcanic rock fragments
can occur in large quantities in some sands, the porosity shown
here could be several porosity units too low. The sonic log was
calibrated to the core porosity in T Fm, but this core is in poor
quality rock. This does not calibrate the higher porosities. No
calibration was possible in A Fm.
Lack
of a uranium corrected gamma ray log (CGR) hampers shale calculations.
The overall high GR readings indicate either uranium salt precipitation
(usually in fractures), feldspathic sands, or other radioactive
rock fragments. It is impossible with this data set to separate
these events from the shale content. Porosity calculations are
suspect because of this.
Log
character and borehole condition indicate a highly stressed, probably
fractured, reservoir.
Results
show many individual sands that probably contain gas. Any one of
these could be leaking through poor cement to surface, or leaking
and charging lower pressure water zones uphole.
Recommendations
The recommended logging program for future wells
is a multi array induction log with SP and GR, a compensated density
neutron log with PEF, GR, and caliper, a natural gamma ray spectral
log, and an array sonic log with compressional, shear, and Stoneley
curves, with GR and caliper. This suite provides sufficient redundancy
to compensate for bad hole conditions, mineral variations, fractures,
and radioactive salts.
A study
should be undertaken to map water resistivity versus depth in the
region, since no RW data was provided for this project.
In
future wells, conventional and special core analysis to obtain
capillary pressure and electrical properties should be contracted
to help calibrate water saturation.
If
possible, available core should be re-analyzed, described, and
special core analysis properties obtained as soon as possible to
allow recalibration of this log analysis.
Respectfully Submitted
E. R. (Ross) Crain, P.Eng.
Consulting Petrophysicist
Petrophysical Analysis Report - Unconventional Reservoir
SAMPLE REPORT #2
24 Month 2014
INTRODUCTION
We were requested by A. Person, P.Geol. of PQR
Resources Inc. to analyze the log and core data over the B Formation
in 3 wells in the subject area.
Final results of the
petrophysical analysis will be used to assist in assessment of
reservoir quality and to assist in stimulation design.
A comprehensive multi-mineral petrophysical analysis was computed
and delivered as electronic images along with this report. Net pay
summaries are included in the body of this report. Rock mechanical
properties were calculated based on reconstructed logs derived from
the petrophysical analysis, for use in stimulation design programs.
The reconstructed logs eliminate gas effect and low quality data
caused by rough borehole.
AVAILABLE DATA
The log suite consisted of density, neutron, PE,
sonic, GR, and resistivity logs. Two wells had crossed-dipole shear
sonic logs and one had a nuclear magnetic resonance log.
No conventional,
side-wall, or shale rock core analysis data were provided. Capillary
pressure data was provided for three samples. Total organic carbon
analysis and X-Ray diffraction mineralogy data was provided for one
well.
ANALYSIS PROCEDURE
Digital log data was
provided by the client. These data were analyzed with a complex
lithology petrophysical model, which accounts for the effects of
heavy minerals and gas, using our proprietary META/LOG analysis
script, running in the PowerLog software package.
TOC and XRD mass
fraction lab measurements were converted to volume fractions based
on the component densities. These were used to calibrate the kerogen
correction to crossplot porosity and to calibrate clay and mineral
volumes in the b-040 -I/094-O-05 well. The parameters and
scale factors derived here were used in the other two wells.
Shale volume was
calculated from the total gamma ray curve using a Clavier
correction. Individual clean and shale lines were chosen for each
zone in each well. Because of the effect of uranium on the total
gamma ray curve, clean and shale lines were adjusted by comparison
with the shale volume calculated from the density-neutron separation
method. The final shale volume was calculated from the average of
the two methods. Results match the clay volume fraction available
from XRD data in well XXX.
Total organic carbon
(TOC) was calculated using the Issler method with resistivity and
density data, and calibrated to the lab data with scale and offset
factors based on the available lab data in well XXX. The log derived
TOC mass fraction matches the available lab data extremely well. The
mass fraction curve was then converted to volume fraction for use in
the porosity calculation.
Porosity was
calculated from the shale corrected complex lithology density
neutron crossplot model. The results from this model are relatively
independent of mineralogy and compensated for gas effects. However,
the effect of kerogen volume is included in this initial result, so
the kerogen volume is subtracted to obtain the final effective
porosity value.
There is no core
porosity data to help calibrate this result. However, there is a
nuclear magnetic log with effective porosity in d-34-K/094-O-05.
This curve matches the calculated effective porosity curve in that
well quite closely. The NMR effective porosity is unaffected by
kerogen and is the best available check on the final effective
porosity in this project.
The PE curve in XXX
well was affected by barite weighted mud. It was reconstructed from
multiple regression based on data in the other two wells.
The dominant
lithology is described as quartz (with clay), some calcite
(increasing somewhat with depth), and minor pyrite. This would need
a three mineral log analysis model since the effect of pyrite on the
lithology calculation can be quite significant. Because of gas
effect, lithology models that use the density or neutron log data
cannot be used, leaving only a two-mineral model based on PE
available.
To account for
pyrite, pyrite volume was derived from a multiple regression using
all available lithology indicating logs, calibrated to the XRD
pyrite volume. This curve was then used to remove the effect of
pyrite from the PE curve, allowing it to be used in a 2-mineral
model.
Lithology was then
calculated with a 2-mineral model using the pyrite corrected PE
data, with a mineral mixture chosen as quartz and calcite. The final
result is a three mineral model with quartz, calcite, and pyrite
(and clay) that matches the XRD data quite well. All TOC and XRD
data points are plotted on the log analysis depth plots for
comparison.
The Simandoux
equation was used for water saturation calculations. This model
reverts to the Archie equation in the clean zones.
Water resistivity was
set at 0.060 ohm-m at 25C for all zones. Electrical properties were
set at A = 1.00, M = N = 1.65. Formation temperature gradient was
set at 3.13C / 100 m with a surface temperature of 10C. This gives a
formation temperature of 86C at 2430 meters.
No lab measured
electrical properties were available; those used are based on prior
experience in tight sands.
A permeability index
was generated from a standard relationship; the equation is Perm =
10^(20.0 * PHIe – 2.0). There is no core data for calibrating this
value so it should be treated as a qualitative guide. The
permeability derived from the Coates equation was provided for the
NMR log in d-34-K. It has been plotted on the depth plot for
comparison to our calculated results. These permeabilities do not
include that from natural fractures or stimulation.
We were requested to
calculate the acoustic anisotropic coefficient of the interval,
based on differences between the X and Y axis crossed-dipole sonic
log data. Even on an expanded scale log, there was no significant
difference between the two log curves. We conclude that the acoustic
anisotropic coefficient (Kani) is zero.
Using the complete
petrophysical analysis results described above, reconstructed log
curves were generated. This step removes bad hole and gas effects
from the logs so that accurate water-filled rock mechanical
properties can be calculated. This process is also used to create
missing log curves where needed.
Calculated mechanical
properties include Biot’s constant, bulk, shear, and Young’s moduli,
and Poisson’s Ratio. A brittleness coefficient (Lame’s constant,
Lambda) was also calculated. These results are displayed, along with
the lithology track, on a separate depth plot.
These results are not
calibrated as there is no lab data available. However, all results
are within normal limits for water-filled rocks of this type and are
suitable for use in stimulation design programs.
Use of the raw log
curves instead of the reconstructed logs should be strongly
discouraged because the gas effects are quite large and will lead to
calculation of erroneous stimulation parameters.
RESULTS
Two sets of net pay flags were generated; one set
used a porosity cutoff of 3% and a saturation cutoff of 50%. The
second used a more lenient set of values, with porosity cutoff of
2.5% and a saturation cutoff of 65%.. A shale volume cutoff of 45%
was used throughout. Both net pay flags are shown on the depth plots
of results. Tables of these results are shown on the next page.
NET PAY SUMMARIES
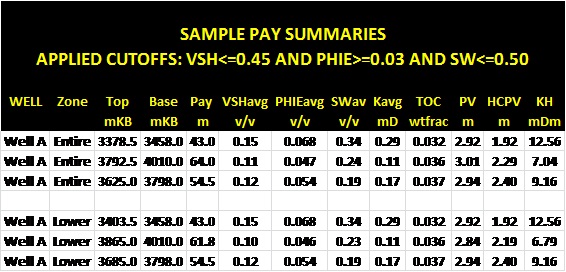
All data in the above
tables are based on measured log depths.
Free gas in place can
be calculated from these data using appropriate gas volume factor,
temperature, pressure, and area.
The Trican report
provided by the client indicated that adsorbed gas volume would be
small. In any case, adsorbed gas volume cannot be calculated from
the log analysis as there is no gas content (Gc) versus total
organic carbon (TOC) relationship available in the data set
provided.
Results of the log
analysis of the wells are contained in the depth plots, LAS files,
and net pay spreadsheet delivered with this report. All depth plots
are measured depth displays.
CONCLUSIONS
Petrophysical analysis using the shale and kerogen
corrected complex lithology model is believed to be reliable for
porosity and saturation, and can be used to determine original free
gas in place. There is insufficient data to calculate adsorbed gas
in place from this petrophysical analysis.
The permeability
index provided in our work should only be used qualitatively.
Mechanical rock
properties calculated from these results are believed to be reliable
and can be used as input to stimulation design software.
Results match
available TOC and XRD data. However, there is no useable porosity or
permeability control data from conventional or sidewall cores.
Confidence in this analysis could be markedly improved if a cored
well was added to the well complement.
Formation tops,
formation names, and perforation intervals were provided by the
client, and were used on our answer and raw data plots for zone
identification purposes only. We express no opinion on the
correctness of the name designations or associated depths.
This is not a
reserves or resource appraisal report.
Respectfully submitted
E. R. (Ross) Crain, P.Eng.
Principal Consultant
Spectrum 2000 Mindware
Disclaimer
All interpretations expressed in this report, and contained in any
attachments thereto, are opinions based on inferences from
geophysical well logs and/or laboratory measurements provided by the
client.
No economic decisions should be made by anyone based solely on the
results or opinions expressed in this report or its attachments. The
reader should exercise prudent business practices along with sound
geological and engineering judgment before any further actions are
undertaken.
Spectrum 2000 Mindware Ltd cannot and does not guarantee the
accuracy or correctness of any interpretations, and we shall not be
liable or responsible for any loss, costs, damages, or expenses
incurred or sustained by anyone resulting from any interpretation
made by our officers, agents, or employees.
We do not represent that this communication, including any files
attached, is free from computer viruses or other faults or defects.
We will not be liable to any person for any loss or damage,
including direct, consequential, or economic loss or damage however
caused, and whether by negligence or otherwise, that may result
directly or indirectly from the receipt or use of this communication
or any files attached to this communication.
Forensic
Petrophysical Report
SAMPLE
REPORT #3
24 Month 2012
Introduction
We were requested
to review the log, core, and production test information provided by
Company B on seven wells in the Dark River
area of Country C. The work was performed for Another One of Noisy
Petroleum Consultants Ltd, team leader of an integrated study to assess
development potential of a deep, tight gas reservoir. Six of the wells
penetrated the gas reservoirs to varying depths and one was an off
structure exploration well (C-1). The six field wells were N-1, 2, 3, 4,
5, and 6. The zone of interest is the P Formation of middle Jurassic age,
between approximately xxxx and xxxx meters below KB.
The P Fm is a thick sand-shale sequence with fluvial braided stream sands
in the upper layers, fluvial channel to terrestrial deposits in the
middle layers, and marine sands in the basal layers. Considerable
volcanic and metamorphic minerals occur in the upper and middle P Fm. The
middle sands are moderately over-pressured and the basal sands even more
so. Basal sands appear to be more continuous than the middle sands. Upper
sands are probably more isolated due to the braided stream environment.
Porosity is typically in the range of 5 to 11 % but permeability seldom
exceeds 0.10 md even in the best sands.
Available Data
Raw data depth
plots of the well logs for the seven wells were provided. These were
re-plots from a log analysis software package and not the original logs.
Typical log suite included gamma ray, SP, caliper, deep and shallow
resistivity, density, neutron, sonic, and PEF (in newer wells). No
spectral gamma ray data was recorded. This would have been very useful in
accounting for the feldspar and other possibly radioactive rock fragments
in the sands.
Log data quality is reasonably good, with more problems from rough hole
conditions in the three older wells (N-6, 2, and C-1). Production test
information was posted on these logs, but the information is incomplete.
It is not always clear which test results belong to which zone as no
depths are recorded here. Perforation depths are contained in other
documents but there was no time available during this phase to assemble
this information for use in reviewing the logs.
Some flow rates or the fact that there may have been no flow is not
consistently noted. Crossflow between zones is evident as some zones
produce more when isolated than when co-mingled with other zones.
A composite log with the same raw data plus petrophysical computed
results, as well as core porosity and permeability, gas mud log curves,
and pressures and permeability from test results were provided. Some of
the core data appears to be from sidewall cores. Core data for N-2 was
analyzed at surface and at overburden conditions and a listing of this
data was provided. No listings for core data in other wells was found but
values are plotted on the composite logs.
Data from tests is mostly after fracturing or acidizing. Test results
were handwritten on these logs and mimic the information posted on the
raw data plots. A graphical presentation of the sample descriptions is
included on this depth plot. In the four newer wells, this is an
excellent data set and correlates well to the log curves. On the three
older wells, the match varies with borehole condition.
A structure map and cross section with the six field wells was provided.
A crossplot of core porosity vs core permeability was provided. Data was
coded by sand quality but the wells or zones included are not listed. It
is not stated whether the data is from whole core, plug, or sidewall
samples. It is probably from N-2.
Thin section petrographic analysis data for N-3, 4, and 5 describe the
mineral composition and visual porosity for a number of samples. In upper
and middle sands, the volcanic rock fragments compose 30 to 60% of the
clastic material. These are termed heavy minerals in log analysis and
must be accounted for in the log analysis model. The exact definition of
which volcanic minerals are present is not given.
No special core electrical properties or capillary pressure data was
provided. No water resistivity or water chemistry for the area was
provided.
Discussion of
Petrophysical Computations
The petrophysical
computation and display of results for five of the seven wells (N-3, 6,
7, 5, and 4) is excellent, with one major problem, discussed below.
The model appears to use gamma ray and density neutron separation as
shale indicators with the minimum of these two methods being used as the
final shale volume.
Porosity is from a shaly sand crossplot of density and neutron data. This
model does not account for heavy minerals, such as volcanic rock
fragments. Since the PEF curve is available on newer wells, it could be
used to generate a heavy mineral correction. Normally, the same result
can be obtained from the density neutron crossplot in a complex lithology
model, but this is not possible (automatically) in a gas zone due to gas
effect masking the heavy mineral effect.
The heavy mineral correction will raise computed porosity compared to the
present values. The correction could add 1 to 4 porosity units depending
on the existing values of density, neutron, heavy mineral content, and shale
volume. Where porosity is low, this is a significant increase in
reservoir volume. Where PEF is not available, a zoned approach using a
density neutron complex lithology model with a forced matrix density
greater than 2.65 gm/cc will achieve similar results.
Since log analysis porosity is significantly less than core porosity in
almost every cored well, this correction should be attempted. As noted
earlier, some of the core data is from sidewall cores, so core porosity
may be a little too high in these cases. The source of the core data
should be ascertained before a final calibration to core is attempted.
Data from the thin section analysis shows some limonite, an iron rich
mineral. This may affect stimulation success and formation damage while
drilling.
Constraints for rough hole effect on the density neutron calculation were
very well done. There are very few spikes or anomalously high porosity
events on these five wells.
In N-7, the sonic log corrected for shale was used for porosity as the
other curves were missing. Results compare favourably to the other four
wells in this group.
Water saturation was computed from a shale corrected model, but there is
no indication of which model or what RW, temperature, or shale properties
were used. The results are reasonable compared to the porosity of these
sands but are quite low when compared to the permeability. This may be
due to highly deformed pores caused by ductile minerals or infilling with
diagenetic minerals. Saturation values will change only slightly if
porosity is increased with the heavy mineral correction.
Existing thin section results would have to be studied further to gain a
better understanding of the porosity-permeability-saturation
relationship. There is insufficient time allocated in this phase for a
thorough review of the thin section data. Further special core studies
are also needed.
Permeability was calculated from a model that varies with the sample
description. It has been calibrated to the core and the plotted log curve
matches the core very precisely. If log analysis porosity is raised with
the heavy mineral correction, this algorithm will have to be adjusted
slightly to retain the excellent fit to the core shown on these wells.
The exact nature of the permeability transform is not mentioned.
A water saturation cutoff was used on these wells to mark pay zones. The
saturation cutoff varies with the sample descriptions in the range 60 to
80%. There is no evidence that a porosity cutoff was used, but it may
have been, as a 5% cutoff was used in N-4. Choosing a net pay cutoff in
tight, deep gas sands is very difficult and may be impossible. The
cutoffs on these plots are satisfactory for identifying zones of
interest, but there is no way to tell at the moment whether they over or under
estimate gas in place.
The log analysis in N-4 could be improved. There is no water saturation
or permeability curve on the plot. The scale of the BVW curve differs
from the PHIe curve, so the visual interpretation of Sw from these curves
is misleading. A 5% porosity cutoff was used to identify interesting
intervals. This is different than the wells described above. This well
should be recomputed with the same model as the previous five. No flows
are reported on the log plots, so this may be a very poor well, but for
gas in place calculations, it needs to be upgraded.
Well C-1 is off structure and has been computed with somewhat different
model and parameters. The shale beds are not shaly enough, so too much
porosity shows in the shaly sands. Too much porosity shows in cleaner
sands where rough hole conditions affect the results. Permeability is
from a different model than other wells and is based on faulty porosity
data. It cannot be used in its present form. Sample descriptions are poor
due to cavings and there is no core data. These problems make it very
difficult to repair this log analysis, but the attempt should be made if
the well is needed for aquifer assessment.
Recommendations
1. Assemble all core
data, classify as to source (sidewall, whole core, plugs),
and review for consistency and usefulness. Re-plot core
porosity vs core permeability. List and compare thin section
visual porosity to core porosity.
2. Summarize thin section lithology breakdown vs depth
to determine the quantity of heavy minerals and feldspar
present.
3. Identify which heavy minerals are present and determine
their grain density and PEF values. Generate the properties
of a generic heavy mineral that is the average of the
minerals identified.
4. Re-compute log analysis with a complex lithology model
using the PEF curve or a zoned RHOMA value to correct
porosity for heavy minerals. Re-compute N-4 and C-1 with
the same attention to rough hole as the other wells.
5. Adjust permeability transform to compensate for this
change in the porosity model.
6. When better electrical properties or cap pressure data
becomes available, recalibrate water saturation model.
7. Run net pay, hydrocarbon pore volume, and flow capacity
summations for each individual sand body (do not co-mingle
zones) using no cutoffs except shale volume < 50%.
8. Plot these sums vs test results (flow rate) on a crossplot
to see if any trend exists. If there is a trend, it will
assist in choosing cutoffs for net pay. Since the permeability
transform appears to match core very well, the final cutoff
may be permeability.
9. In new wells, add the gamma ray spectral log to the
logging suite. This will allow a better shale volume calculation
and help distinguish feldspathic sands from shaly sands.
It will also eliminate the false indication of shale caused
by uranium salts in the sands.
10. Additional core should be taken in new wells to cover
representative sand bodies from all environments, particularly
those with volcanic rock fragments as a major component.
11. Existing core and chip samples should be re-described,
and new core and chip samples should be adequately described,
to determine which volcanic minerals are present and in
what quantities.
12. Special core analysis to determine electrical properties,
capillary pressure, and relative permeability should be
performed on existing and new core in cores from each
depositional environment. This is needed to calibrate
initial water saturation and residual gas saturation.
13. New cores should be viewed with SEM and thin section
petrography to determine the pore geometry that leads
to such low permeability and low water saturation in moderate
porosity.
Respectfully Submitted
E. R. (Ross) Crain, P.Eng.
Consulting
Petrophysicist
Research
Petrophysics Report
SAMPLE REPORT #4
24 Month 2012
Introduction
We were requested to review the
log and pressure test data on eight wells and to
perform an independent petrophysical and overpressure
analysis.
The
interval of interest is from sea floor to the top
of Chalk or top of Zechstein evaporites if Chalk
is not present. The main pay zones are the Montrose
sands lying above the Chalk.
The
objective of this project is to evaluate the efficacy
of the standard overpressure indicator method based
on sonic log trend line analysis. The approach
is commonly known as the Eaton method, but similar
discussions have been published many years earlier.
Available Data
Log and pressure data for this project
was provided in digital form. Logs consisted of resistivity,
sonic, gamma ray, and caliper over most of the interval,
and density neutron over lower portions of some wells.
Sonic data was missing in one well and had a large
gap in another.
Formation
pressure data for the Montrose were provided for
six wells.
A
report from the client was provided, which contained
discussion and results of their analysis using
the Eaton method on a number of wells.
Method
Digital log curves, pressure data, and
formation tops for the wells were provided by the client.
These were entered into Spectrum 2000 Mindware Ltd's
proprietary log analysis program called Meta/Log. All
log and pressure data were converted to metric units.
Data recorded inside casing was eliminated and some editing
was done to remove spikes.
Shale
volume was determined from the gamma ray log. Porosity
was determined by the sonic log corrected for shale.
The density neutron crossplot porosity was also
calculated where possible. No water saturation
calculation was made. The equations used were:
Neutron
porosity
PHIN = NPHI : fraction
Density
Porosity
PHID = (RHOB-2.65)/(2.65-1.00) : fraction
Sonic
Porosity
PHIS = (DELT-182)/(656-182) : fraction
Shale
Volume
Vsh = MIN(1,MAX(0,((GR-GRcl)/(GRsh-GRcl)))) : fraction
Effective
Porosity
PHIe = MIN(0.3*(1-VSH),MAX(0,0.5*(PHIN-VSH*0.28+PHID-VSH*0.05))) :fraction
GRcl
and GRsh were chosen uniquely for each well.
These
results were used to determine shale beds suitable
for analysis of overpressure by the Eaton method.
Data below the zone of interest (Montrose) was
deleted from the working files after this analysis
step.
The
calculation steps for the Eaton method are listed
below:
Actual
shale travel time
DELTsh = IF(VSH>0.5,DELT,100) : us/m
Normal
shale travel time compaction trend line
DTnorm = 10^(Log(3.281*175)-((DEPTH/3000)*(Log(175*3.28)-Log(100*3.28)))) :us/m
Difference
between actual and normal sonic values
DTdiff = MAX(0,+DELTSH-DTNORM) : us/m
Overburden
pressure
SOV = (Ln(DEPTH-EKB)-0.5185)/3.47 : gm/cc
Shale
Pore Pressure as a gradient
SPP = SOV-(SOV-1)*(MIN(1,DTNORM/DELT))^3 : gm/cc
Shale
pore pressure as head of water
SPP-M = (SPP-1)*(DEPTH-EKB) : head in meters
Shale
pore pressure as a pressure
PRESsh = 9.81*(SPP-M+DEPTH-EKB) : KPa
RFT
pressure from lookup table
RFTPRES = VLOOKUP(DEPTH,PRESSURE_TABLE,2)*6.89 : KPa
RFT
pressure as a head of water
RFTHEAD = MAX(0,-DEPTH+EKB+RFTPRES/9.81) : head in meters
DTnorm
is the sonic trend line chosen in a shallow shale
zone to represent the normal compaction trend.
The position and slope of this line is very subjective.
The line finally chosen is very similar to the
line used by the client. My first pick fits the
sonic log better but gave less overpressure than
my final pick. There is, in fact, very little valid
sonic data in the shallow sequence to which a line
can be fitted. Depth plots of both my initial and
final lines, along with the sonic log curves for
7 wells, are provided under separate cover. The
final line was picked to account for actual mud
weights used to maintain the holes and to approximate
actual Montrose reservoir pressures at the top
of the gas/oil column.
SOV
is the overburden stress. This equation varies
from place to place. It was supplied by the client
and is assumed to be suitable for this region of
the North Sea. SPP is the shale pore pressure from
the Eaton equation. It is converted to meters of
head of water (SPP-M) and to pressure in KPa (PRESsh).
For comparison, the RFT pressures for any depth
were found in a lookup table (psi) and converted
to head in meters and pressure in KPa.
Depth
plots at 1:10,000 scale were made of all these
results plus the raw log data. A lithology track
was created from the Vsh curve and a depth function
related to the formation name. Thus sandstone,
limestone (chalk), anhydrite, and salt were shown
where appropriate.
Results
Results are contained in the depth plots
supplied under separate cover. There is little difference
between this work and the client's work. We have added
pressure vs depth curves to the plots as these are
sometimes easier to visualize than head of water curves.
The
final compaction trend line was chosen as a compromise.
The initial choice generated very little overpressure,
yet mud weight data supplied by the client suggested
higher pressure results were needed to account
for the mud weights actually used. The final choice
was arrived at after several iterations. The final
trend gives shale overpressure values close to
actual mud weight gradients and close to actual
formation pressures at the top of the Montrose
structure.
Matching
the actual Montrose pressure is not a requirement
of the method. A normally pressured shale is sufficient
to act as a seal, even for the relatively high
buoyancy caused by the large oil and gas column.
It should be noted that none of the Montrose data
shows significant overpressure in the reservoir.
The pressures are close to those expected for the
hydrocarbon buoyancy.
Conclusions
There are many unknowns and assumptions
in log analysis for overpressure. These include the
subjective nature of the normal compaction trend line,
the lack of control on parameters in the SOV and SPP
equations, and the variable silt content within the
shale itself.
The
effect of a gas phase in porosity within the silt
component of the shale cannot be accounted for,
even if it were known to be present. Invasion by
drilling fluid removes most of the gas from the
region seen by the sonic log, so the effect should
be very small. A well log model study could be
undertaken to assess the magnitude of gas effect.
Gas leaking through fractures would probably not
influence this method. If the other unknowns described
in the previous paragraph could be calibrated,
it is unlikely that gas in the silt would pose
additional problems, but the model study suggested
above would quantify this.
It
should be noted that the seismic signal may be
influenced by gas in porosity in the silty shales
or in fractures. Seismic studies for detection
of overpressure may be compromised by this effect,
while the sonic log is not.
The
validity of the Eaton method for calculation of
shale pore pressure has not been proven, since
there are no actual pressure data points within
the shale interval that can be used for calibration.
Recommendations
Results from the Eaton method should
be used only as an indicator of possible overpressured
shales.
Results
should not be used as a quantitative measure of
the amount of overpressure.
Further
work is required from this specific area to validate
the overburden stress (SOV) formula, on which the
Eaton method depends.
Pressures
must be acquired from stray sands within the overpressured
shales to calibrate the terms in the Eaton equation
for SPP and to validate the normal compaction curve
(DTnorm) for use in this specific area..
There
is no reason to believe that the parameters in
these equations are universal constants and they
need confirmation from this area to be used reliably
in this area.
Respectfully Submitted
E.
R. (Ross) Crain, P.Eng.
Consulting
Petrophysicist
|