Saturation basics
Water
saturation is the ratio of water volume to pore volume. Water
bound to the shale is not included, so shale corrections must
be performed if shale is present. We calculate water saturation
from the effective porosity and the resistivity log. Hydrocarbon
saturation is 1 (one) minus the water saturation.
Most
oil and gas reservoirs are water wet; water coats the surface
of each rock grain. A few reservoirs are oil wet, with oil on
the rock surface and water contained in the pores, surrounded by
oil. Some reservoirs are partially oil wet. Oil wet reservoirs
are very poor producers as it is difficult to get the oil to
detach itself from the rock surface. It is fairly easy to take a
core sample, clean it and dry it, then make the rock oil wet.
However, reservoir rocks are seldom clean and dry, so that same
rock in-situ will often be water wet.
A water wet reservoir (left)
An oil wet reservoir (right)
In a
water wet reservoir, the water is held in place by surface tension.
The surface water does not move while the oil or gas is being
produced. This situation is shown below (left).
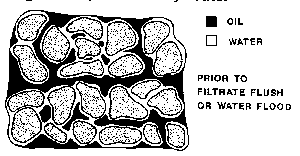 |
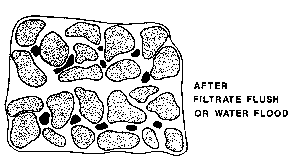 |
Water wet formation with hydrocarbon before invasion (left)
and after invasion (right).
The same illustrations are used to describe a reservoir at
initial conditions (left) and after production by aquifer
drive or an efficient water flood - water moves in to
replace the oil that is withdrawn (right). |
When
a reservoir is drilled, some of the fluids near the wellbore are
pushed away and the zone is invaded by the drilling fluid. If
hydrocarbons were present, the water saturation after invasion
will be higher than the original reservoir conditions. A shallow resistivity log will see the invaded zone
water saturation. A deep resistivity log should see the original
formation water saturation as long as invasion was not too deep.
Production of oil or gas will often change the water saturation, but
the amount of change varies with the drive mechanism.
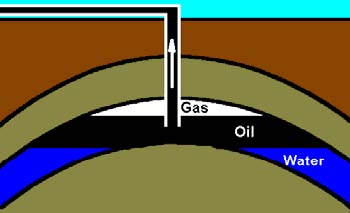
Aquifer drive (left) pushes oil up, increases water saturation as
the oil is produced. Gas cap drive (right) pushes oil down, but
water saturation does not change until the gas that replaced the oil
is also produced. If there is no aquifer, both situations produce
only by expansion drive - in this case water saturation does not
change unless a water flood is imposed by the field operator.
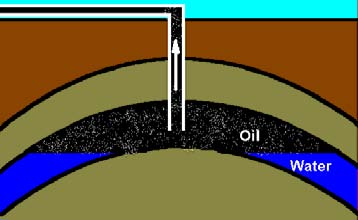
Expansion drive is also called solution drive as it is the gas in
solution in the oil that pushes oil out of the reservoir. Water
saturation does not change and oil recovery is very small (5 to 10%
depending on gas-oil ratio and oil viscosity) unless a water flood
is instituted. Gas reservoirs can produce with reasonably high
recovery from pure expansion drive (Sw nearly constant), but there
may also be an aquifer drive component (Sw will increase over time).
Reservoir monitoring
is used to assess the changes in water saturation over time.
Monitoring is accomplished by periodically running appropriate logs
through casing and analyzing the logs for porosity and water
saturation. Changes in the position of the oil-water or gas-oil
contact can lead to a workover of the well to restrict the
perforated interval to reduce water or gas production. Modern
technology applied to older wells may even find bypassed pay zones
to find ways to improve the economic performance of the well.
basic Saturation MODEL -
ARCHIE'S METHOD
Almost
all saturation computation methods rely on work originally done
by Gus Archie in 1940-41. He found from laboratory studies that,
in a shale free, water filled rock, the Formation Factor (F) was
a constant defined by:
1: F = R0 / RW
He
also found that F varied with porosity:
2:
F = A / (PHIt ^ M)
For
a tank of water, R0 = RW. Therefore F = 1. Since PHIt = 1, then
A must also be 1.0 and M can have any value. If porosity is zero,
F is infinite and both A and M can have any value. However, for
real rocks, both A and M vary with grain size, sorting, and rock
texture. The normal range for A is 0.5 to 1.5 and for M is 1.7
to about 3.2. Archie used A = 1 and M = 2. In fine vuggy rock,
M can be as high as 7.0 with a correspondingly low value for A.
In fractures, M can be as low as 1.1. In some rocks, M varies
with porosity.
Note that R0 is also spelled
Ro in the literature.
For
shale free rocks with both hydrocarbon and water in the pores,
he also defined the term Formation Resistivity Index (I) as:
3:
I = Rt / R0
4: Sw = (1 / I) ^ (1 / N)
Archie
used an N of 2 and the usual range is from 1.3 to 2.6, depending
on rock texture. It is often taken to equal M, but this is not
supported by core data in all cases. A, M, and N are called the
electrical properties of the rock. They are found usually from
laboratory measurements.
Rearrangement
of these four equations gives the more usual Archie water saturation
equation:
5:
SWa = (A * RW@FT / (PHIe ^ M) / RESD) ^ (1 / N)
True
resistivity (Rt) is estimated from the deep resistivity log (RESD)
or RESD corrected for borehole effects and invasion (RESDc). RESD
can come from many different logging tools, such as the Electrical
Survey (64 inch Normal), induction log, laterolog, and all their
modern siblings. The actual curve name and abbreviation must be
determined from the log heading
Porosity
is determined from any one of a dozen available methods.
Shale
corrections are applied by adding a shale conductivity term with
an associated shale porosity and shale formation factor relationship.
Numerous authors have explored this approach, leading to numerous
potential solutions for water saturation
Definition of Saturation
Saturation of any given fluid in a pore space is the ratio of
the volume of that fluid to the pore space volume. For example,
a water saturation of 10% means that 1/10 of the pore space is
filled with water; the balance is filled with something else (oil,
gas, air, etc. - a pore cannot be “empty”). As for
porosity, saturation data is often reported in percentage units
but is always a fraction in equations.
Porosity
is the capacity of the rock to hold fluids. Saturation is the
fraction of this capacity that actually holds any particular fluid.
Porosity, hydrocarbon saturation, the thickness of the reservoir
rock and the real extent of the reservoir determine the total
hydrocarbon volume in place. Hydrocarbon volume, recovery factor,
and production rate establish the economic potential of the reservoir.
Irreducible
water saturation (SWir) is the minimum water saturation obtainable in
a rock. Water is usually the wetting fluid in oil or gas reservoirs,
so a film of water covers each pore surface. The surface area
thus defines the irreducible water saturation. Formations at irreducible
water saturation cannot produce water until water encroaches into
the reservoir after some oil or gas has been withdrawn. Small
pores have larger surface area relative to their volume so the
irreducible water saturation is higher. If pores are small enough,
the irreducible water saturation may be 1.0, leaving no room for
oil or gas to accumulate.
The
initial water saturation (SWi) is the saturation of an undisturbed
reservoir with no prior production from any earlier well. Usually
SWir = SWi, at least above the oil water transition zone. In the
transition zone, SWa is higher than SWir and some water would be
produced if the well was completed in this interval.
In a
reservoir that has had some production, SWa may be higher than SWir
(and higher than SWi) so some water may be produced with the oil.
Of
the total amount of oil or gas present in a reservoir, only a
fraction of it can be produced, depending on the recovery efficiency.
This recovery factor, normally determined by experience, is typically
in the 20% to 50% range for oil, and may be as high as 95% for
gas zones, or as low as 5% in heavy oil. Recovery factor can sometimes
be estimated from log data by observing the moveable hydrocarbon
volume.
Here
are the standard definitions needed to understand this Chapter.
DFN
11: |
Total
water saturation (SWt) is the ratio of |
|
-
total water volume (BVW + CBW) to |
|
-
total porosity (PHIt) |
|
1:
SWt = (BVW + CBW) / PHIt |
DFN
12: |
Effective
water saturation (SWe) is the ratio of: |
|
-
free water volume (BVW) to |
|
-
effective porosity (PHIe) |
|
2:
SWe = BVW / PHIe |
This
is the standard definition of “water saturation”.
Older books use this term to define total water saturation. Since
all interpretation methods described here correct for the effects
of shale, we are not normally interested in the total water saturation,
except as a mathematical by-product. As effective porosity approaches
zero, the water saturation approaches one (by edict, if not by
calculus)
DFN
13: |
Useful
water saturation (SWuse) is the ratio of: |
|
-
useful water volume (BVW - BVI) to |
|
-
useful porosity (PHIuse) |
|
3:
SWuse = (BVW - BVI) / PHIuse
|
DFN
14: |
Irreducible
water saturation (SWir) is the ratio of: |
|
-
immobile or irreducible water volume (BVI) to |
|
-
effective porosity (PHIe) |
|
4:
SWir = BVI / PHIe
|
DFN
15: |
Residual
oil saturation (Sor) is the ratio of: |
|
-
immobile oil volume (BVHr) to |
|
-
effective porosity (PHIe) |
|
5:
Sor = BVHr / PHIe
|
DFN
16: |
The
water saturation in the flushed zone (Sxo) is the ratio of
: |
|
-
free water in the flushed zone, to |
|
-
effective porosity, which is assumed to be the same porosity
as in the un-invaded zone. |
|
6:
Sxo = BVWflushed / PHIe |
The
amount of free water in the invaded zone is usually higher than
in the un-invaded zone, when oil or gas is present. Thus Sxo >=
Swe. The water saturation in the invaded zone between the flushed
and un-invaded zone is seldom used.
DFN
17: |
Further
constraints that should be remembered are: |
|
7:
PHIt >= PHIe >= PHIuse |
|
8:
SWt >= SWe >= SWuse. |
|
9:
PHIt = PHIe when Vsh = 0 |
|
10:
SWt = SWe when Vsh = 0 |
All
volumes defined above are in fractional units. In tables or reports,
log analysis results are often converted to percentages by multiplying
fractional units by 100.
|