X-RAY FLUORESCENCE
BASICS (XRF)
X-ray fluorescence (XRF) is the emission of
characteristic "secondary" (or fluorescent) X-rays from a
material that has been excited by bombarding it with high-energy
X-rays or gamma rays. The phenomenon is widely used for
elemental analysis of rocks and minerals.
The work is usually done in a laboratory along with other
petrographic assessments. Used mostly to log cores, it can also
be used on individual rock samples. Handheld and portable core
logging versions are available for use at the wellsite.
Because XRF can give quantitative values for the elements in
a rock, it is often used to help evaluate the results from
elemental capture spectroscopy (ECS) logs. The elemental
composition from XRF in the lab or ECS in the wellbore can be
inverted to a mineral composition using a least squares inversion
algorithm.
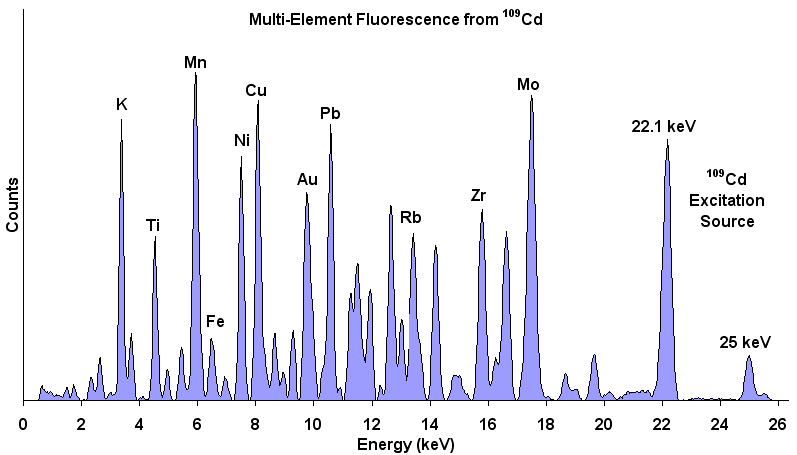
XRF Energy spectrum of a material showing energy peaks for
specific elements in the sample. The relative amplitudes
indicate the relative concentration of each element in the
material. A non-negative least squares inversion can transform
element concentrations into mineral weight percent.
The range of elements that can be observed varies with the
design of the instrument. A typical handheld can only recognize
the elements between Magnesium and Lithium, for example. A full
scale lab model can handle all the way to Uranium.
X-RAY FLUORESCENCE
EXAMPLE
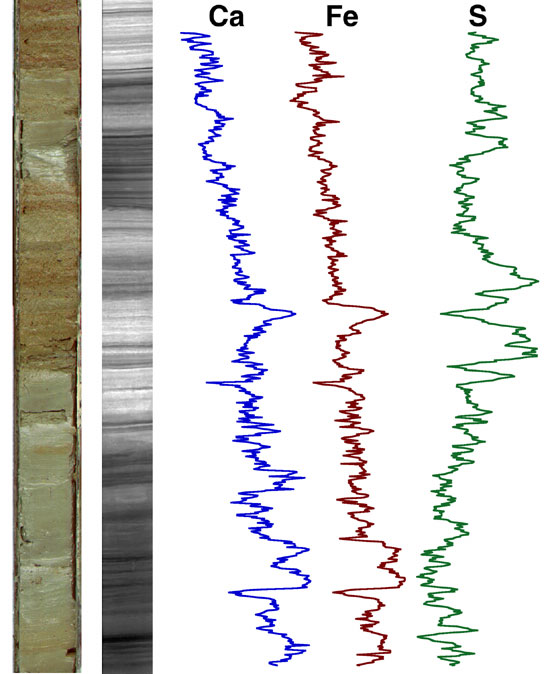
Example of an XRF log taken on a core (core photo at
left). (image: Woods Hole Oceanographic)
HOW
X-RAY FLUORESCENCE
WORKS
Source
Wikipedia
When materials are
exposed to short-wavelength X-rays or to gamma rays, ionization
of their component atoms may take place. Ionization consists of
the ejection of one or more electrons from the atom, and may
occur if the atom is exposed to radiation with an energy greater
than its ionization potential. X-rays and gamma rays can be
energetic enough to expel tightly held electrons from the inner
orbitals of the atom. The removal of an electron in this way
makes the electronic structure of the atom unstable, and
electrons in higher orbitals "fall" into the lower orbital to
fill the hole left behind. In falling, energy is released in the
form of a photon, the energy of which is equal to the energy
difference of the two orbitals involved. Thus, the material
emits radiation, which has energy characteristic of the atoms
present. The term fluorescence is applied to phenomena in which
the absorption of radiation of a specific energy results in the
re-emission of radiation of a different energy (generally
lower).
Each
element has electronic orbitals of characteristic energy.
Following removal of an inner electron by an energetic photon
provided by a primary radiation source, an electron from an
outer shell drops into its place. There are a limited number of
ways in which this can happen. The main transitions are given
names: an L→K transition is traditionally called Kα, an M→K
transition is called Kβ, an M→L transition is called Lα, and so
on. Each of these transitions yields a fluorescent photon with a
characteristic energy equal to the difference in energy of the
initial and final orbital. The wavelength of this fluorescent
radiation can be calculated from Planck's Law:
1: Lambda = h * c / E
Where:
h = Planck's constant
c = speed of light in a vacuum
E = energy difference
Lambda = wavelength of emitted photon
The fluorescent radiation can be
analyzed either by sorting the energies of the photons
(energy-dispersive analysis or EDXRF) or by separating the
wavelengths of the radiation (wavelength-dispersive analysis or
WDXRF). The latter technique is no longer in widespread use as the
advances in computer and detector technology favour the energy
dispersive method.
Once sorted, the intensity of each characteristic radiation is
directly related to the amount of each element in the material.
|