WHAT IS RESERVOIR DESCRIPTION?
Reservoir description, sometimes called reservoir characterization
or reservoir modeling, attempts to create a static and a dynamic
three-dimensional description of an oil or gas reservoir, based on
the one- and two-dimensional data from well bores and seismic
surveys. A good reservoir description costs money and requires an
integrated, multi-discipline approach.
The
direct aims of the static reservoir description are:
1. Extrapolate core data to uncored wells
2. Define quantity and distribution of porosity, saturation, and
permeability in each well
3. Interpolate rock property data between wells
4. Identify flow units from porosity vs permeability populations
5. Build a knowledge base that evolves with the reservoir
development
The direct aims of the dynamic reservoir description are:
1. Test the static model for accuracy by matching production
history
2. Predict future performance under various operational scenarios
3. Optimize production for maximum long-term economic return
A large
fraction of the data for the static model comes from the
petrophysical analysis, along with the core and petrographic data
used to calibrate the log analysis.
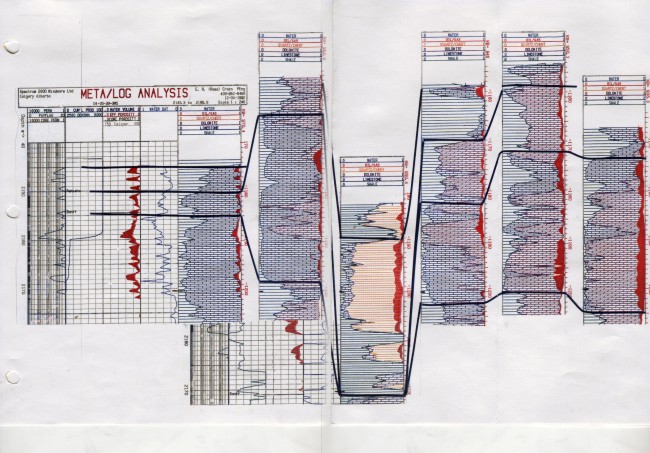
The beginnings of a reservoir description that
will continue to evolve over time. During an earlier
iteration, the sand channel in the middle of the carbonate reservoir
had not been discovered, so
the earlier model had to be completely revised.
Reservoir Description means many things to many people. Thousands of
consultants, contractors, and oil company department heads use the
term with subtle or serious differences in meaning.
The
petrographer thinks reservoir description means defining the pore
geometry, pore size, and pore throat radius distributions, and
reservoir mineralogy, using thin section analysis, X-ray diffraction
(XRD), and scanning electron microscopes (SEM). The modern term used
is “porosity imaging”. The source material is drill cuttings or
samples from cores. Their work is usually at the sub-millimeter
level.
Core
analysts think reservoir description is the measurement of porosity,
permeability, grain density, capillary pressure, relative
permeability, and electrical properties of the rocks, as well as
facies description from observation of the depositional environment
seen in slabbed core and core photographs. This work is at the
centimeter level.
Petrophysicists see reservoir description as the evaluation of well
logs to obtain reservoir rock and fluid properties, such as shale
volume, porosity, water saturation, permeability, and lithology on a
foot by foot basis, as well as sums and averages over specific
reservoir units. This work is usually calibrated by core analysis
and petrographic data where it is available. When well log data is
combined with other geoscience data to form a coherent picture, it
is called Integrated Petrophysics. Some work, such as analysis of
formation microscanner images, may be at the centimeter level, but
most is done at the tool resolution level – usually 0.3 to 1 meter.
Geophysicists see reservoir description as the creation and mapping
of seismic attributes or inverted seismic data to illuminate
variations in reservoir properties between well control. This work
is at the multi-meter level vertically and horizontally, but
provides finer spatial resolution than logs and cores, which are
dictated by well spacing. Attributes are usually calibrated to
petrophysical log analysis results.
Geologists perceive reservoir description as the interpretation and
mapping of petrographic results, core analysis, petrophysical rock
properties, and seismic attributes into stratigraphic sequences
and/or flow units. In the simplest cases, the mapping is based
solely on correlation of raw log curves. In more elaborate studies,
all of the measured and computed data will be mapped. Dipmeter and
pressure transient analysis results may be introduced to assist in
correlation or definition of reservoir boundaries or fault planes.
These maps are also at the multi-meter level vertically and either
the seismic shot point spacing or well spacing areally. The
geological model obtained is the static reservoir description which,
of course, can be monitored and varied over time by acquisition of
new petrology, core data, or petrophysical rock properties from new
wells or logs run through casing. Time-lapse (4-D) seismic or
through casing logs may also
be used to monitor hydrocarbon contact changes.
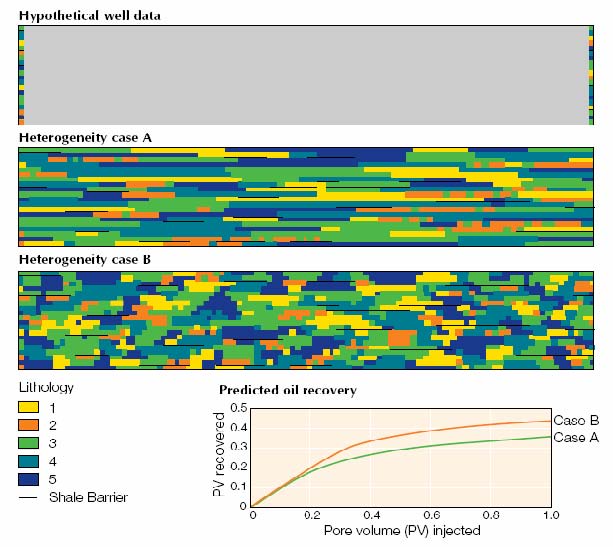
Different geostatistical realizations of well log data suggest
different hydrocarbon recoveries.
Reservoir engineers view reservoir description as the analysis and interpretation
of pressure transient data from drill stem tests or
production tests. Flow capacity (permeability times thickness) and
distance to reservoir boundaries (if they are close enough to be
sensed by the pressure response) are the usual results obtained. In
addition, pressure changes with production versus time provide grist
for the material balance calculation mill. Production history (oil,
gas, and water volumes versus time) coupled with decline curve
analysis leads to predictions of ultimate hydrocarbon recovery. This
work is also at the multi-meter level vertically and limited to the
tested or produced wells only. Reservoir engineers are concerned
with this dynamic reservoir description, as well as the static
description for calculating reservoir volume and recoverable
reserves.
Simulation engineers visualize reservoir description as a totally
dynamic description of reservoir performance. They do use the static
reservoir description (the geological model) as the basic foundation
for the reservoir simulation. The bricks and mortar added to this
foundation are the pressure and production data, and fluid
properties, from the reservoir engineer. The object of the
simulation is first to match production history, then to predict
future behaviour of the reservoir. A good history match usually
means that the geological model is reasonably accurate. The critical
test is to compare the performance prediction with actual
performance after a few years has elapsed. The reservoir simulation
grid is usually a few to many meters vertically and many meters
areally. Since more than one geological model could provide an
adequate history match, calibration can only come with the passage
of time and the arrival of new well data. Various production
scenarios may be run in order to optimize reservoir recovery and
economics.
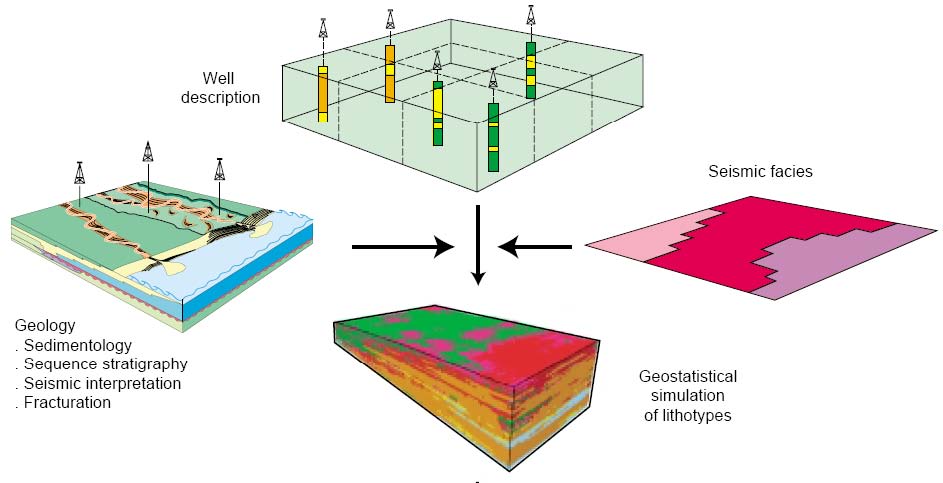
Integrating the various geoscience disciplines is central to
achieving a reasonable dynamic reservoir description.
Production and facilities engineers see the dynamic reservoir model as a template
for design and economic evaluation of production, gathering,
treating, and pipeline equipment required to handle the predicted
reservoir performance. The timing of compressor installations, water
disposal wells, and conversion of wells to injection are paramount
considerations. Both undersized and oversized facilities reduce the
economic return from hydrocarbon production.
Drilling and production engineers use the various scenarios to plan
in-fill drilling and re-completion operations on the wells in the
reservoir.
Economic engineers use the dynamic and static models to predict cash
flow, financing requirements, and investment decisions. Management
and shareholders use the models to assist in making decisions as
well.